Breaking Barriers:The Past, Present and Future of Focused Ultrasound and Diffuse Intrinsic Pontine Glioma
Images
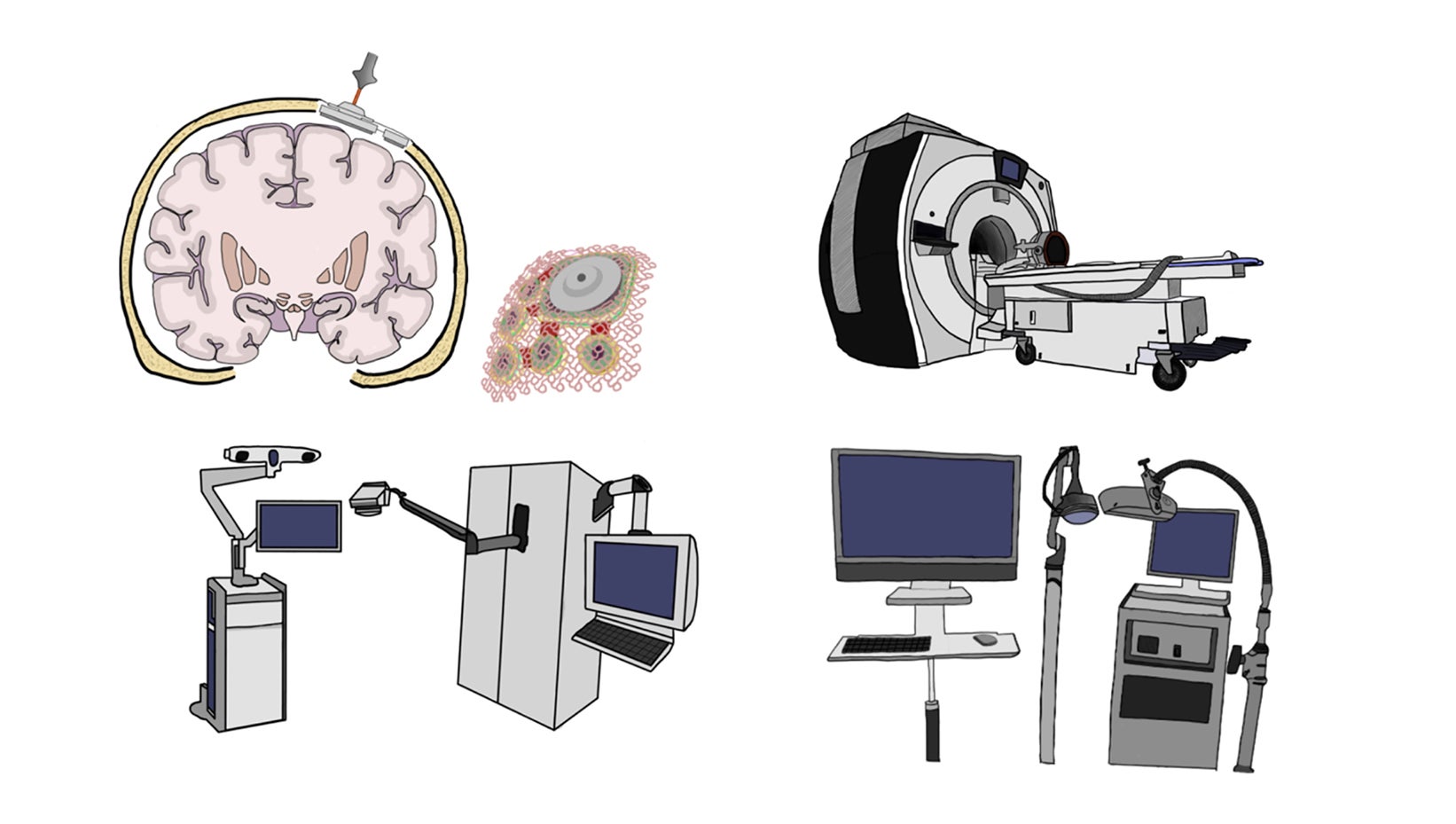
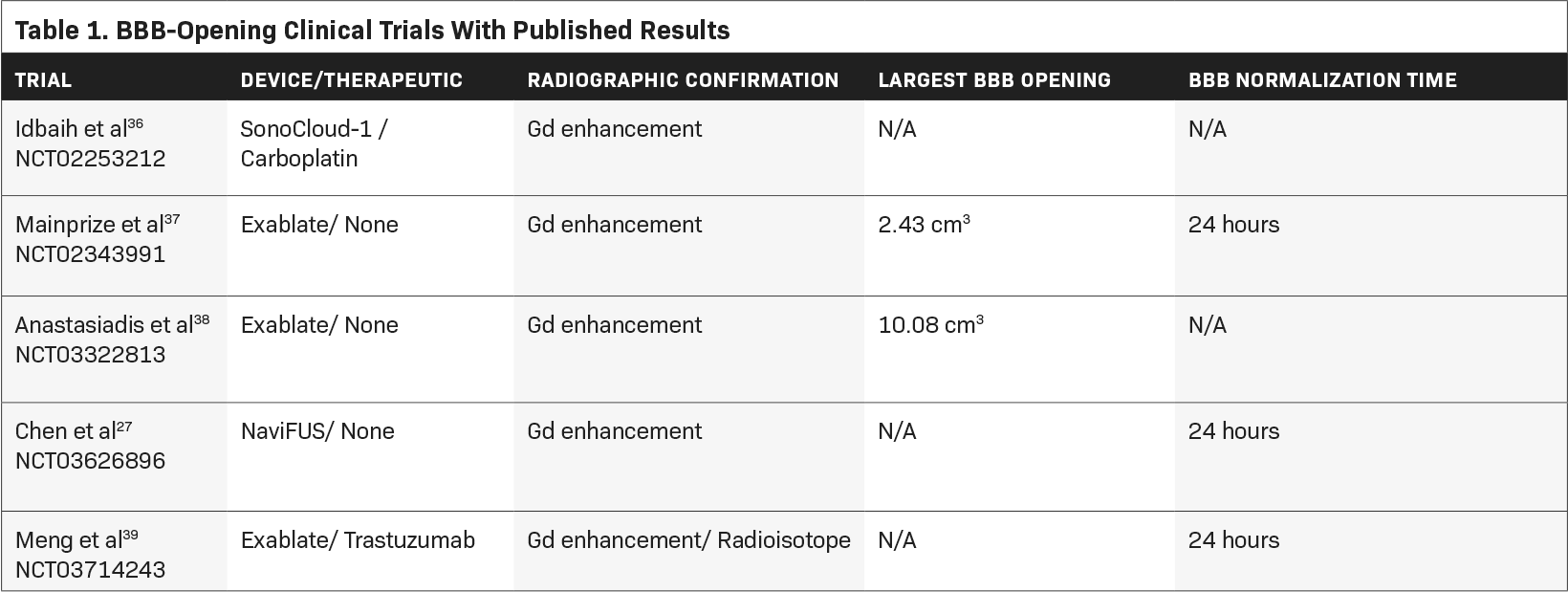
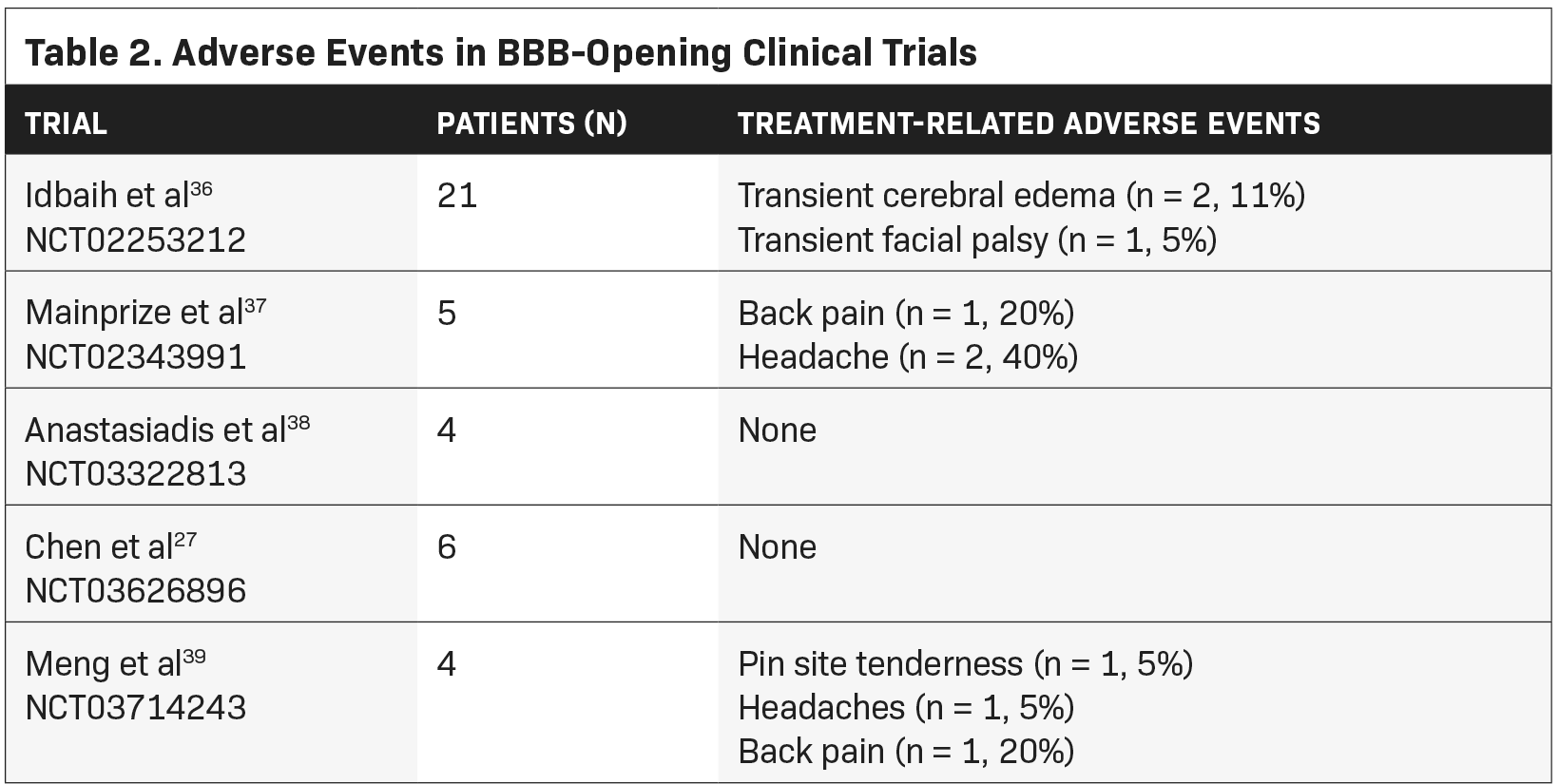
SA-CME credits are available for this article here.
Abstract
Diffuse intrinsic pontine glioma (DIPG) is a malignant childhood tumor of the brainstem with a dismal prognosis. While recent progress has been made in understanding the molecular underpinnings of the disease, the blood-brain barrier (BBB) remains a significant blockade for effective drug delivery. Low-frequency focused ultrasound (FUS) therapy in conjunction with intravenous microbubbles can transiently disrupt the BBB in a localized manner to facilitate drug delivery. This is achieved through stable cavitation, the process by which oscillations in bubble volume induce minor mechanical stress at the cellular level, disrupting endothelial tight junctions and leading to increased BBB permeability. Here we review preclinical studies performed over the last decade that have evaluated the safety and feasibility of FUS-mediated BBB opening in the brainstem. Furthermore, we cover the published phase 0-II clinical trials of low-frequency FUS therapy in the adult glioma population, as well as the phase I clinical trials in DIPG that are underway.
Keywords: DIPG; diffuse intrinsic pontine glioma; brainstem glioma; convection-enhanced delivery; focused ultrasound
Introduction to Focused Ultrasound and DIPG
Focused Ultrasound
Since it was first described by brothers Pierre and Jacques Curie in the late 1800s, the piezoelectric effect — the generation of electricity by crystals under mechanical stress — has been leveraged for remarkable technological achievements. The first application was seen in World War I with the development of sonar devices by the French government to detect submarines.1 In 1935, Johannes Gruetzmacher fit a curved lens on the end of a piezoelectric generator and found that ultrasound waves could be focused.2,3 In the late 1940s, the first attempt at clinical ultrasonography in the diagnosis of brain tumors ultimately failed due to the high density of the skull. However, 1 year later George Ludwig made great progress by imaging gallstones across the abdomen and advancing research into the interactions between ultrasound waves and soft tissues. While this work was occurring, other researchers attempted to utilize ultrasound technology for therapeutic effects by focusing the acoustic energy to heat and lesion tissue. This was first reported in animals by Lynn and Miller at Columbia University in 1942 and advanced by others such as Ballantine at Massachusetts General Hospital and the Fry brothers in Indianapolis.4 Therapeutic ultrasound now encompasses a wide variety of categories including thermal ablation, histotripsy, nerve stimulation, and the opening of the blood-brain barrier (BBB).
The desired bioeffect of ultrasound depends both on the acoustic parameters of the waves as well as the characteristics of the target tissue. Generally, there are thermal and nonthermal effects, but all involve some degree of mechanical alteration induced by the waves’ energy.5 Thermal effects secondary to high-frequency FUS are achieved by simple absorption of ultrasonic energy by the tissue. The sustained exposure to higher intensities produces heat and leads to irreversible tissue injury. This mechanism is well established and can be employed for tumor ablation, clot lysis, and intracranial lesioning for pathologies such as essential tremor,6 Parkinson’s disease, chronic intractable neuropathic pain and obsessive-compulsive disorder.7,8 Lower-frequency FUS exhibits its effects through nonablative, nonthermal mechanisms. With a lower frequency and thus a smaller amount of acoustic energy directed at the targeted area, the threshold to produce ablative lesions is not met. Instead, a mechanical effect of low-intensity FUS can be achieved through interactions with intravenously delivered gases, which can ultimately lead to disruption of the BBB.
BBB
The BBB is a highly discriminatory barrier between the central nervous system (CNS) and its vasculature. The structure of the BBB includes astrocyte foot processes and specialized endothelial cells. Unique to the cerebrovasculature, these endothelial cells form a continuous barrier, anchored to each other by tight junctions, which prevents the intercellular passage of material into the CNS. Additionally, there are fewer fenestrations and a variety of efflux pumps, which help protect the CNS from potentially harmful substances in the blood stream, but also prevent the passage of therapeutics into the brain.9 Although the BBB is protective, transiently and focally increasing the permeability of the BBB has been a target for investigation. BBB disruption could allow for the delivery of drugs like chemotherapy, immunotherapy, stem cells, and gene therapy to CNS targets.10 To improve penetration, investigators have explored changes in drug molecular size and lipophilicity, as well as carriers that can cross the BBB.11,12 Current attempts to disrupt the BBB have shown moderate success but with potentially significant adverse effects: direct injection of drugs through convection-enhanced delivery is invasive and carries risks of damage to neural tissue; and the use of hypertonic solutions, such as mannitol, to osmotically induce BBB opening, leads to a nonselective, global increase in BBB permeability that can cause unwanted drug-induced CNS toxicity.13-15 Violating the BBB in a safe, targeted, and transient manner using noninvasive sound delivery is a unique application of FUS and has the potential to change the medical management of infiltrative brain tumors.
FUS and BBB
Investigations of the nonthermal effects of FUS have been underway for decades.16 The success of FUS in BBB disruption is largely achieved through the process of cavitation and the resultant mechanical stress on the endothelium. With the application of ultrasonic waves, injectable gaseous microbubbles within the cerebrovasculature absorb the energy and begin to oscillate.17 This oscillation is known as acoustic cavitation and can be inertial or stable. Inertial cavitation is a violent implosion or explosion of the microbubbles that leads to tissue destruction. Stable cavitation on the other hand is the process by which these oscillations in volume induce minor mechanical stress at the cellular level, disrupting endothelial tight junctions and leading to increased BBB permeability.18 The continuous expansion and compression of the microbubbles is transmitted to the vessel wall as mechanical stress, separating the endothelial lining. The oscillation also induces jet streaming, or acoustic streaming, which adds further stress to the vessel wall.19 Passage through the temporarily disrupted BBB is thought to be through 4 mechanisms: widening of interendothelial spaces due to opening of tight junctions, upregulation of transcytosis as evidenced by increased cytoplasmic vesicles, channel and fenestration formation, and free movement across the injured endothelium.20
DIPG
DIPG is a malignant tumor that arises from the brainstem primarily in children ages 5 to 9. It is a difficult disease carrying a mean life expectancy of less than a year, with less than 10% of patients surviving more than 2 years.21 Given that these tumors grow diffusely through the pons, one of the most highly eloquent regions of the brain, surgical resection is not feasible.22 The mainstay of treatment for this disease is fractionated radiation therapy, which is the only treatment modality that has been shown to prolong survival in these patients.23 Many trials have been conducted to evaluate different combinations of chemotherapy without success. Over the last decade, significant advancements have been made in understanding the molecular characteristics of these tumors. Nevertheless, there remains little success with these targeted therapies in clinical trials. The BBB, as described above, is thought to be a major factor in limiting the penetrance of drugs to these infiltrative brain tumors. Furthermore, there is very minimal tumor-induced BBB disruption in these patients as evidence by the absence of contrast enhancement on MRI in the majority of cases. Thus, FUS appears to be a promising option for the treatment of this highly malignant cancer.
FUS — Current Devices
Three classes of FUS devices are under investigation for use in humans: fixed-frame MRI-guided devices (MRgFUS), implantable ultrasound devices, and frameless neuronavigation-guided devices24 (Figure 1).
Exablate
One of the first FDA-approved devices was the Exablate system by Insightec, an MRgFUS machine initially developed to treat uterine fibroids by thermal ablation.7 The technology was adapted, and the newer Exablate Neuro system has been FDA-approved for thalamotomy and is in use in more than 10 clinical trials investigating its ability to disrupt the BBB. The device consists of a helmet transducer that includes more than 1000 elements coordinated to transmit ultrasound to a precise target. The treatment planning and monitoring is performed using real-time MRI guidance. Treatment with the device requires placement of a stereotactic head frame with fixed skull pins. The extended duration of the procedures coupled with the discomfort of lying in a fixed frame within an MRI machine can be a limiting factor.
SonoCloud
The SonoCloud-9, developed by the French company Carthera, is an implantable, unfocused, ultrasound system that features 9 transducers. The device is placed within the skull bone following a craniotomy, which can occur either during a scheduled brain tumor resection, or as a standalone ambulatory procedure. While the noninvasive systems require mathematical calculations to overcome the complexities of achieving effective sonication through bone, the SonoCloud bypasses the skull and sits directly above the dura. As the investigators on the original clinical trial note, the absence of bone-induced attenuation of the US waves eliminates the need for intraprocedure MRI monitoring.25 The SonoCloud-9 recently received an FDA breakthrough device designation and is under investigation in multiple clinical trials.
NaviFUS and TheraWave
The most recently developed devices rely on neuronavigation tracking, a technology commonly used for many neurosurgical procedures. Using infrared cameras, the precise location of FUS transducer placement can be achieved by registering the coordinate system developed from the preprocedural MRI or CT with the coordinates of the patient’s skull. There are 2 such devices in varying stages of development: the NaviFUS System, developed by a group in Taiwan, and a similar device developed by our group at Columbia University.26,27 Like the SonoCloud, these neuronavigation-based devices have largely been explored with low-frequency ultrasound and BBB disruption.28 The NaviFUS device has been used in multiple clinical trials investigating its efficacy in the treatment of both glioblastoma (GBM) and drug-resistant epilepsy.29 The Columbia device (TheraWave) has demonstrated targeted and reproducible BBB disruption in several nonhuman primate studies. It is currently classified under an FDA investigational device exemption and has been registered in a clinical trial for Alzheimer’s disease and another for DIPG (discussed below). The advantages to these systems are the avoidance of invasive procedures and the absence of prolonged time in the MRI scanner. These are lightweight, portable devices that can be used in an outpatient setting, allowing for potentially wider access and application.
Preclinical Work
The past decade has seen a significant increase in preclinical FUS-mediated BBB-opening studies, with several groups narrowing their focus on drug delivery to the brainstem. In 2018, Alli and colleagues from the Hynynen lab at the University of Toronto demonstrated the safety of doxorubicin delivery to the murine brainstem following ultrasound treatment.30 Using an animal MRgFUS device, BBB opening was achieved and confirmed both with contrast-enhanced MRI and Evans blue staining. Using liquid chromatography/mass spectrometry (LCMS), significantly higher doses of doxorubicin were found in the brainstem of mice that underwent sonication. This group also found that treatment was not associated with any cardiopulmonary or motor deficits.
During the same year, the Chen lab from Washington University in St. Louis published 2 papers on the delivery of nanoparticles to the pons. The first study evaluated the use of FUS in the presence of intravenous, radiolabeled gold nanoclusters.31 In contrast to the previous study, which used contrast-enhancement as a marker of BBB disruption, this project allowed for a more precise, real-time tracking of drug delivery via in vivo microPET/CT imaging of64 Cu-integrated gold nanoclusters. Additionally, the spatiotemporal distribution of these nanoclusters was then quantified by imaging at different time points postsonication. In their follow-up study, Ye and colleagues attempted to curtail the systemic drug exposure by utilizing intranasal delivery.32 They successfully demonstrated less systemic uptake but equivocal pontine distribution with radiolabeled gold nanoclusters following inhaled intranasal delivery in mice. In their most recent preclinical study, using a RCAS/tv mouse DIPG model, Zhang et al demonstrated a twofold increased uptake of64Cu-Cu nanoclusters in the murine tumors that were exposed to FUS compared with the nontreated tumors. In a follow-up study, Ye et al evaluated the ability of FUS to enhance the delivery of immune checkpoint inhibitor therapy in a murine brainstem glioma model.33 Using an intranasally delivered programmed cell death-ligand 1 antibody (aPD-L1) tagged with a fluorescent dye, they demonstrated an approximately fourfold increase in drug concentration following FUS therapy compared with intranasal delivery alone.
Building on these studies, our group set out to investigate the safety of FUS-mediated chemotherapy delivery in a preclinical pontine glioma model.34 We implanted high-grade glioma cells into the pons of immunocompetent mice. Once tumor formation was identified on MRI, we treated mice with FUS and found no significant cardiopulmonary or motor deficits associated with treatment. Histological analysis did not show any harmful effects. Furthermore, using LCMS, a fivefold increase in the concentration of etoposide in mice that underwent FUS was noted compared with mice treated with etoposide alone. Our study also found that multiple FUS treatments were not associated with any negative effects.
Ishida et al published similar experiments using doxorubicin but in immunocompromised mice with patient-derived xenografts.35 While the tumor model was more accurate, the absence of immune response in this model was a limitation. The justification behind using doxorubicin was rooted in several in vitro studies demonstrating its efficacy in treating multiple DIPG cell lines, plus its known limited ability to cross the BBB. These authors similarly found a fourfold increase in drug concentration within the mouse tumors that underwent FUS compared with the control group. Additionally, they found decreased tumor growth rate on MRI and decreased ki67 — a marker of tumor proliferation — on immunohistochemical staining in the treated mice. Nevertheless, there was no survival benefit in the study, which the authors attributed to the limited single treatment plan as well as the systemic toxicity of doxorubicin. Future animal studies will need to optimize drug formulations to avoid systemic toxicity, while incorporating a more robust FUS regimen that can increase tumor exposure to higher drug concentrations over a prolonged period.
Clinical Trials
BBB Opening Trials in Adult Glioma
Multiple published clinical trials have investigated the utility of FUS-mediated BBB opening in the adult supratentorial glioma popula- tion (Tables 1 and 2 summarize the clinical trials and serious adverse events, respectively). The first published trial came from the Carpentier group at the Pitie Salpetriere University Hospital using the SonoCloud CarThera device.25,36 In a single-arm, phase 1/2a study, 19 patients were implanted and treated with the CarThera device alongside the delivery of carboplatin. A total of 65 treatments were delivered, more than 50 of which demonstrated evidence of BBB disruption on MRI. This radiographic effect was quantified based on the degree of contrast enhancement and correlated with amount of acoustic pressure. They found an increased delivery of energy associated with a more robust BBB opening. No significant adverse events (SAEs) were observed. One patient experienced a transient facial nerve palsy, which resolved within 2 hours followed by the administration of steroids. Several patients also experienced transient brain edema that resolved with steroids. Although the study was underpowered, patients who underwent radiographic BBB breakdown lived longer than those who did not (OS 12.94 months vs 8.64 months).
In 2019, Mainprize et al published the first clinical trial evaluating the safety of noninvasive FUS and drug delivery in brain tumor patients.37 In this phase 1, single-arm study, 5 patients with GBM underwent MRgFUS treatment with concurrent chemotherapy (temozolamide or doxorubicin) 1 day prior to surgical resection. No adverse clinical or radiographic events were observed. To quantify radiographic BBB opening, they measured the percentage of sonicated tissue that exhibited contrast enhancement (increased signal intensity compared with the nontreated adjacent brain tissue), the highest of which was 50%. Four of the 5 patients had evidence of contrast enhancement following treatment. In 2 of the patients, chemotherapy levels were quantified with LCMS. The authors found a trend toward increased drug concentration in the treated tissue compared with the nontreated tissue. A phase 0 study published in Proceedings of the National Academy of Sciences of the United States of America (PNAS) 2021 similarly evaluated the safety of MRgFUS in 4 adult patients with lobar diffuse glioma.38 They did not observe any SAEs with treatment, although no therapeutic was delivered. Furthermore, histological analysis of treated tissue did not demonstrate any necrosis or microhemorrhage. Each treatment volume was measured, the largest of which was 10.08 cm3< . Using intravenously administered fluorescein, they found a 2.2-fold increase in drug accumulation in the brain tissue that underwent FUS-mediated BBB opening, compared with nontreated tissue.
In Taiwan, Chen et al reported their findings in a 6-patient, phase 1 pilot study. They performed a dose escalation design with the NaviFUS Neuronavigation-guided focused ultrasound system device, without drug delivery, to determine a safe energy level for BBB disruption.27 No SAEs were observed. Similar to the Carpentier study, they found a correlation between degree of signal change on MRI and the amount of acoustic energy delivered. All radiographic changes were transient and returned to near baseline at 24 hours. Interestingly, they found a lack of significant immunological response on histological analysis 1 week following treatment.
Meng et al published their phase 1, FUS-mediated drug-delivery study in brain tumor patients, specifically in those with Her2-positive breast cancer and brain metastases.39 Four patients were treated with MRgFUS and radiolabeled trastuzumab, a monoclonal antibody for the Her2 receptor. No treatment-related serious adverse events were observed. Unlike prior studies that relied on contrast-enhanced, T1-weighted MRI, single-photon emission computerized tomography (SPECT) imaging was used to track radiotracer uptake. Following MRgFUS and intravenous injection of 111In-BzDTPA-NLS-trastuzumab, increased SPECT signal intensity was observed only in regions in which FUS was targeted, but not in other metastatic lesions that weren’t sonicated. This was the first study to demonstrate real-time tracking of drug delivery to brain tumors following BBB opening.
Current and Future Trials in DIPGs
There are 3 current trials in the DIPG patient population. Our group began the first phase 1 clinical trial (NCT04804709) in children with recurrent diffuse midline glioma. Study participants underwent treatment with FUS combined with oral panobinostat. The purpose of the study was to evaluate the feasibility of opening the BBB safely in 1, 2, or 3 tumor sites. The trial followed a 3+3 Number of Tumor Sites (NOTS) escalation scheme, which refers to the number of openings in the BBB. Subjects started the first cycle of the treatment arm with 1 tumor site and moved on to incrementing NOTS levels if no dose-limiting toxicities (DLTs) were observed.
Another trial (NCT05123534) has recently opened at Children’s National Hospital, University of California San Francisco (UCSF), and the Ivy Brain Tumor Center using sonodynamic therapy, a technology that utilizes ultrasound-generated light, or sonoluminescence. This light can then trigger the byproduct of an injectable therapy, SONOALA-001, to activate cell death exclusively within glioma cells. This is a phase 1/2 study in newly diagnosed DIPG following radiation therapy using a dose escalation model with both drug dose and energy delivered. Patients are administered SONOALA-001 and sonicated using the Exablate Neuro system several hours later. This treatment modality is also being studied in adult GBM patients in a clinical trial in Arizona, and early reports have noted its safety. Most recently, a single-arm, nonrandomized, prospective feasibility study was opened at Children’s National Hospital to treat DIPG patients with FUS and doxorubicin using the Exablate Neuro system (NCT05630209). This study is recruiting as of press time.
Other Applications – Liquid Biopsy
The first liquid biopsy study following FUS was performed by the Chen lab. Using an enhanced green fluorescent protein (eGFP)-transduced GBM cell line, Zhu et al observed a significant increase in plasma eGFP mRNA in multiple preclinical mouse models using a variety of acoustic pressures.40 Meng et al published the first study in humans to evaluate the feasibility of liquid biopsy following treatment with ultrasound.41 In 9 patients undergoing clinical trial for GBM with Exablate, blood samples were collected before and after sonication. Non-brain-tumor patients undergoing FUS alone were used as controls. They found an increase in cell-free DNA (cfDNA) as well as neuron-derived vesicles and brain derived proteins associated with the treatment. They also found methylation signatures within the cfDNA samples following BBB opening that were distinct from the cfDNA collected pre-BBB opening. Interestingly, for the 1 patient in the trial with an IDH1 mutant glioma, they observed a two- to threefold increase in IDH1 mutant cfDNA following BBB opening. These findings altogether are very early but demonstrate the feasibility of using FUS-mediated BBB opening as a tool for both drug delivery and noninvasive diagnostics.
Conclusion
Despite recent advancements in the molecular understanding of DIPG, the BBB remains a significant challenge for advancing care in this disease. The advent of FUS-mediated BBB opening has led to an exciting new era in the field of neuro-oncology. Preclinical studies have demonstrated the efficacy of increasing drug distribution within the brainstem and brainstem tumors using FUS. Multiple successful phase 1 clinical trials have been reported in the adult glioma population, and several are underway for those with DIPG. Although it is still early, our hope is that FUS will provide a platform for delivering cutting-edge therapies to improve outcomes for this population.
References
- Man K, Sabourin VM, Gandhi CD, Carmel PW, Prestigiacomo CJ. Pierre Curie: the anonymous neurosurgical contributor. Neurosurg Focus. 2015;39(1):E7. doi:10.3171/2015.4.FOCUS15102
- Gruetzmacher J. Piezoelectric crystal with ultrasonic convergence. Z Phys. 1935;96(5-6):342-349. doi:Doi 10.1007/Bf01343865
- Harary M, Segar DJ, Huang KT, Tafel IJ, Valdes PA, Cosgrove GR. Focused ultrasound in neurosurgery: a historical perspective. Neurosurg Focus. 2018;44(2):E2. doi:10.3171/2017.11.FOCUS17586
- Ballantine HT, Hueter TF, Nauta WJH, Sosa DM. Focal destruction of nervous tissue by focused ultrasound - biophysical factors influencing its application. J Exp Med. 1956;104(3):337-360. doi:DOI 10.1084/jem.104.3.337
- Miller DL, Smith NB, Bailey MR, et al. Overview of therapeutic ultrasound applications and safety considerations. J Ultras Med. 2012;31(4):623-634. doi:DOI 10.7863/ jum.2012.31.4.623
- Elias WJ, Lipsman N, Ondo WG, et al. A randomized trial of focused ultrasound thalamotomy for essential tremor. New Engl J Med. 2016;375(8):730-739. doi:10.1056/NEJMoa1600159
- Abe K, Taira T. Focused ultrasound treatment, present and future. Neurol Med Chir (Tokyo). 2017;57(8):386-391. doi:10.2176/nmc.ra.2017-0024
- Lee EJ, Fomenko A, Lozano AM. Magnetic resonance-guided focused ultrasound: current status and future perspectives in thermal ablation and blood-brain barrier opening. J Korean Neurosurg Soc. 2019;62(1):10-26. doi:10.3340/jkns.2018.0180
- Burgess A, Shah K, Hough O, Hynynen K. Focused ultrasound-mediated drug delivery through the blood-brain barrier. Expert Rev Neurother. 2015;15(5):477-491. doi: 10.1586/14737175.2015.1028369
- Beccaria K, Sabbagh A, de Groot J, Canney M, Carpentier A, Heimberger AB. Blood-brain barrier opening with low intensity pulsed ultrasound for immune modula- tion and immune therapeutic delivery to CNS tumors. J Neurooncol. 2021;151(1):65-73. doi:10.1007/s11060-020-03425-8
- Johnsen KB, Gudbergsson JM, Skov MN, Pilgaard L, Moos T, Duroux M. A comprehensive overview of exosomes as drug delivery vehicles - endogenous nanocarriers for targeted cancer therapy. Biochim Biophys Acta. 2014;1846(1):75-87. doi:10.1016/j.bbcan.2014.04.005
- Pardridge WM. Blood-brain barrier and delivery of protein and gene therapeutics to brain. Front Aging Neurosci. 2019;11:373. doi:10.3389/fnagi.2019.00373
- Doolittle ND, Miner ME, Hall WA, et al. Safety and efficacy of a multicenter study using intraarterial chemotherapy in conjunction with osmotic opening of the blood-brain barrier for the treatment of patients with malignant brain tumors. Cancer. 2000;88(3):637-647. doi:10.1002/(sici)1097-0142(20000201)88:3<637::aid-cncr22>3.0.co;2-y
- Kroll RA, Neuwelt EA. Outwitting the blood-brain barrier for therapeutic purposes: osmotic opening and other means. Neurosurgery. 1998;42(5):1083-99; discussion 1099-1100. doi:10.1097/00006123-199805000-00082
- Mitrasinovic S, Appelboom G, Detappe A, Sander Connolly E, Jr. Focused ultrasound to transiently disrupt the blood brain barrier. J Clin Neurosci. 2016;28:187-189. doi:10.1016/j.jocn.2015.12.011
- Bakay L, Ballantine HT, Jr., Hueter TF, Sosa D. Ultrasonically produced changes in the blood-brain barrier. AMA Arch Neurol Psychiatry. 1956;76(5):457-467. doi:10.1001/archneurpsyc.1956.02330290001001
- Burgess MT, Apostolakis I, Konofagou EE. Power cavitation-guided blood-brain barrier opening with focused ultrasound and microbubbles. Phys Med Biol. 15 2018;63(6):065009. doi:10.1088/1361-6560/aab05c
- Shang X, Wang P, Liu Y, Zhang Z, Xue Y. Mechanism of low-frequency ultrasound in opening blood-tumor barrier by tight junction. J Mol Neurosci. 2011;43(3):364- 369. doi:10.1007/s12031-010-9451-9
- Caskey CF, Stieger SM, Qin S, Dayton PA, Ferrara KW. Direct observations of ultrasound microbubble contrast agent interaction with the microvessel wall. J Acoust Soc Am. 2007;122(2):1191-200. doi:10.1121/1.2747204
- Sheikov N, McDannold N, Vykhodtseva N, Jolesz F, Hynynen K. Cellular mechanisms of the blood-brain barrier opening induced by ultrasound in presence of micro-bubbles. Ultrasound Med Biol. 2004;30(7):979-89. doi:10.1016/j.ultrasmedbio.2004.04.010
- Rashed WM, Maher E, Adel M, Saber O, Zaghloul MS. Pediatric diffuse intrinsic pontine glioma: where do we stand? Cancer Metastasis Rev. Dec 2019;38(4):759-770. doi:10.1007/s10555-019-09824-2
- Warren KE. Diffuse intrinsic pontine glioma: poised for progress. Front Oncol. 2012;2:205. doi:10.3389/fonc.2012.00205
- Haas-Kogan DA, Banerjee A, Poussaint TY, et al. Phase II trial of tipifarnib and radiation in children with newly diagnosed diffuse intrinsic pontine gliomas. Neuro Oncol. 2011;13(3):298-306. doi:10.1093/neuonc/noq202
- Chen KT, Wei KC, Liu HL. Theranostic strategy of focused ultrasound induced blood-brain barrier opening for CNS disease treatment. Front Pharmacol. 2019;10:86. doi:10.3389/fphar.2019.00086
- Carpentier A, Canney M, Vignot A, et al. Clinical trial of blood-brain barrier disruption by pulsed ultrasound. Sci Transl Med. 2016;8(343):343re2. doi:10.1126/sci- translmed.aaf6086
- Pouliopoulos AN, Wu SY, Burgess MT, Karakatsani ME, Kamimura HAS, Konofagou EE. A clinical system for non-invasive blood-brain barrier opening using a neuronavigation-guided single-element focused ultrasound transducer. Ultrasound Med Biol. 2020;46(1):73-89. doi:10.1016/j.ultrasmedbio.2019.09.010
- Chen KT, Chai WY, Lin YJ, et al. Neuronavigation-guided focused ultrasound for transcranial blood-brain barrier opening and immunostimulation in brain tumors.
- Sci Adv. 2021;7(6)doi:10.1126/sciadv.abd0772
- Wu SY, Aurup C, Sanchez CS, et al. Efficient blood-brain barrier opening in primates with neuronavigation-guided ultrasound and real-time acoustic mapping. Sci Rep. 2018;8(1):7978. doi:10.1038/s41598-018-25904-9
- Lee CC, Chou CC, Hsiao FJ, et al. Pilot study of focused ultrasound for drug-resistant epilepsy. Epilepsia. 2022;63(1):162-175. doi:10.1111/epi.17105
- Alli S, Figueiredo CA, Golbourn B, et al. Brainstem blood brain barrier disruption using focused ultrasound: a demonstration of feasibility and enhanced doxorubicin delivery. J Control Release. 2018;281:29-41. doi:10.1016/j.jconrel.2018.05.005
- Ye D, Sultan D, Zhang X, et al. Focused ultrasound-enabled delivery of radiolabeled nanoclusters to the pons. J Control Release. 2018;283:143-150. doi:10.1016/j. jconrel.2018.05.039
- Ye D, Zhang X, Yue Y, et al. Focused ultrasound combined with microbubble-mediated intranasal delivery of gold nanoclusters to the brain. J Control Release. 2018;286:145-153. doi:10.1016/j.jconrel.2018.07.020
- Ye D, Yuan J, Yue Y, Rubin JB, Chen H. Focused ultrasound-enhanced delivery of intranasally administered anti-programmed cell death-ligand 1 antibody to an intracranial murine glioma model. Pharmaceutics. 2021;13(2)doi:10.3390/pharmaceutics13020190
- Englander ZK, Wei HJ, Pouliopoulos AN, et al. Focused ultrasound mediated blood-brain barrier opening is safe and feasible in a murine pontine glioma model. Sci Rep. 2021;11(1):6521. doi:10.1038/s41598-021-85180-y
- Ishida J, Alli S, Bondoc A, et al. MRI-guided focused ultrasound enhances drug delivery in experimental diffuse intrinsic pontine glioma.
- Idbaih A, Canney M, Belin L, et al. Safety and feasibility of repeated and transient blood-brain barrier disruption by pulsed ultrasound in patients with recurrent glioblastoma. Clin Cancer Res. 2019;25(13):3793-3801. doi:10.1158/1078-0432.CCR-18-3643
- Mainprize T, Lipsman N, Huang Y, et al. Blood-brain barrier opening in primary brain tumors with non-invasive MR-guided focused ultrasound: a clinical safety and feasibility study. Sci Rep. 2019;9(1):321. doi:10.1038/s41598-018-36340-0
- Anastasiadis P, Gandhi D, Guo Y, et al. Localized blood-brain barrier opening in infiltrating gliomas with MRI-guided acoustic emissions-controlled focused ultrasound. Proc Natl Acad Sci U S A. 2021;118(37). doi:10.1073/pnas.2103280118
- Meng Y, Reilly RM, Pezo RC, et al. MR-guided focused ultrasound enhances delivery of trastuzumab to Her2-positive brain metastases. Sci Transl Med. 2021;13(615):eabj4011. doi:10.1126/scitranslmed.abj4011
- Zhu L, Cheng G, Ye D, et al. Focused ultrasound-enabled brain tumor liquid biopsy.Sci Rep. 2018;8(1):6553. doi:10.1038/s41598-018-24516-7
- Meng Y, Pople CB, Suppiah S, et al. MR-guided focused ultrasound liquid biopsy enriches circulating biomarkers in patients with brain tumors. Neuro Oncol. 2021;23(10):1789-1797. doi:10.1093/neuonc/noab057
Citation
Englander ZK, Troy C, Tazhibi M, Yoh N, MD; Wei HJ, Feldstein N, Konofagou E, Szalontay L, Wu CC. Breaking Barriers:The Past, Present and Future of Focused Ultrasound and Diffuse Intrinsic Pontine Glioma. Appl Radiat Oncol. 2022;(4):7-13.
December 23, 2022