Dose Painting With the Gamma Knife Lightning Dose Optimizer: Technical Description and Validation of Dose Delivery
Affiliations
- Department of Radiation Oncology, University of Washington – Fred Hutchinson Cancer Center, Seattle
Abstract
Objective:
The recently introduced Gamma Knife (GK) Lightning (Elekta) fast inverse planning dose optimizer allows concurrent optimization of multiple targets, but the optimizer’s use for generating a simultaneous integrated boost (SIB) plan has not been described and validated for accuracy of dose delivery. Here, we describe a method for creating an SIB using the GK Lightning optimizer and conduct validation of dose delivery.
Materials and Methods:
Radiochromic film was positioned in an anthropomorphic phantom. A 15.7-cm3 irregular contour was drawn to represent a brain metastasis resection cavity, a uniform 2-mm radial-expansion contour created, and a 1.6-cm3 contour drawn representing a nodule of residual disease within the cavity. Targets were prescribed 3 Gy (2-mm expansion), 4 Gy (cavity), and 5 Gy (residual disease) in 1 fraction. Within the GammaPlan Lightning optimizer, “beam-on time” and “low-dose” settings were iteratively adjusted to create a clinically acceptable plan. Treatment was delivered using the GK Icon system. The film was scanned and calibrated for absolute dosimetry. Global gamma index analyses were performed at various dose and distance tolerances.
Results:
An 18-minute treatment plan with 40 shots was delivered. Prescription isodose lines were 3 Gy at 55% (2-mm expansion), 4 Gy at 69% (resection cavity), and 5 Gy at 75% (residual disease). All target volumes had greater than or equal to 99% prescription dose coverage and the maximum dose was 6.9 Gy. Paddick conformality indices were 0.79 (2-mm expansion), 0.74 (resection cavity), and 0.15 (residual disease). Gamma index pass rate, mean, and median values were 77%, 0.68, and 0.54 at 1%/1-mm tolerance, 85%, 0.58, and 0.49 at 2%/1-mm tolerance, and 97%, 0.34, and 0.28 at 2%/2-mm tolerance.
Conclusion:
We successfully created an SIB plan with the GK Lightning optimizer, verifying dose delivery within clinically acceptable tolerances. Future work is needed to determine optimal dose levels for use in clinical practice and determine what disease entities may benefit from an SIB.
Introduction
Postoperative radiation therapy is strongly recommended for patients with resected brain metastases (BM).1 Radiographic evidence of residual or recurrent disease is detected in 4% to 13% of patients with resected BM, including in 5% of patients following gross total resection.2 - 5 Such patients may benefit from a simultaneous integrated boost (SIB) to areas of gross disease to improve local control,6 while respecting brain dose-volume tolerances for radiosurgery.
As previous versions of the Gamma Knife (GK) (Elekta) treatment planning system did not explicitly allow for an SIB, Grossberg et al7 described 2 methods of dose painting an SIB. These planning techniques rely on manual forward planning of nested targets, require significant user expertise, are limited to 2 dose levels, do not allow concurrent calculation of dosimetric coverage statistics for the nested treatment volumes, and have not undergone quality assurance for dose delivery validation. Validation of accurate dose delivery is particularly important given the complexity of overlapping dose calculation matrices.8 - 10
Recently, a fast inverse planning (FIP) dose optimizer (Lightning) was introduced for GK, allowing concurrent optimization of multiple targets while incorporating organs-at-risk (OAR) dose constraints.11 This FIP optimizer demonstrated the potential to improve treatment planning quality and efficiency for a variety of clinical scenarios, showing particular utility when treating irregularly shaped targets.12 - 15 Here, we describe the use of the Lightning FIP optimizer for dose painting in GK radiosurgery, using the example of residual or recurrent tumor at the time of adjuvant radiation therapy for a resected BM, and we conduct quality assurance of dose delivery. To demonstrate the accessibility and flexibility of this SIB technique, we extend the 2-target example of Grossberg et al7 (resection cavity and gross tumor) by adding a third nested target consisting of a resection cavity radial margin.16
Materials and Methods
Technical Description of Dose Painting Technique
Standard GK techniques are used for patient setup, image co-registration, and target contouring. At least 2 nested target volumes are created consisting of the resection cavity and/or a radial expansion to a resection cavity margin, plus a volume capturing recurrent or residual gross tumor. Overlapping dose calculation matrices are placed for the nested targets. A prescription dose (in Gy) is specified for each target volume. If desired, a maximum dose can be specified and the option of “full coverage” can be selected to increase target coverage from the default of greater than or equal to 95% to greater than or equal to 99%. The OAR dose constraints can be specified but are not required. An initial plan is then optimized, typically using the default “low dose” (LD) and “beam-on time” (BOT) penalty settings of 0.50 (range, 0.00–1.00).
The initial plan is reviewed for metrics such as target coverage, total treatment time, Paddick conformity index (PCI), gradient index (GI), normal tissue dosimetry, and isodose. While the Lightning FIP does not allow the user to prescribe a specific isodose, the optimized isodose can be manipulated in subsequent iterations by changing the LD and BOT penalty settings, toggling the option of “full coverage,” and/or providing values for “maximum dose.” Treatment plan optimization is iterated under a variety of settings until a clinically acceptable plan is developed, at which point treatment delivery follows standard GK techniques.
Quality Assurance of Dose Delivery
To conduct quality assurance of this technique, we created a clinically representative treatment plan for an anthropomorphic phantom. Using a CT simulation scan of the phantom, a 15.7-cm3 irregular contour was drawn to represent a brain metastasis resection cavity of a recently treated patient. A 2-mm uniform radial expansion was created for an additional contour (25.5 cm3 ). A 1.6-cm3 contour was drawn within the resection cavity to represent a gross tumor nodule. In GammaPlan software v11.3.1, overlapping dose matrices were placed using default positions ( Figure 1 ). The 3 targets were prescribed 3 Gy (2-mm expansion), 4 Gy (resection cavity), and 5 Gy (gross tumor) in a single fraction. Prescription doses were selected to match the radiochromic film’s optimal dose range, while approximating fractional doses used in 5-fraction treatments. Within the FIP optimizer, “full coverage” was selected, no maximum dose or OAR constraints were specified, and LD and BOT penalty settings were iteratively adjusted to create a clinically acceptable plan. On the GK Icon system, a custom occipital mold was made, an unexposed film (Gafchromic RTQA2) placed in the axial plane of the phantom’s cranium, cone-beam CT (CBCT) co-registration conducted, and treatment delivered. The film was scanned (Epson Expression 11000XL) and calibrated for absolute dosimetry using a calibration curve generated from a 6-MV linear accelerator. Corrections were not made for dose delivered by CBCT.17 To evaluate treatment accuracy, global gamma index analyses were performed at various dose and distance tolerances, excluding points below 30% of the maximum dose. The passing rate was defined as the percentage of points with gamma index less than 1. Film dosimetry and analysis were performed using Radiochromic.com software v4.0.18
Axial ( A ), coronal ( B ), and sagittal ( C ) views of a simultaneous integrated boost Gamma Knife treatment plan for an anthropomorphic phantom with 3 nested target contours (red = 2-mm margin, orange = resection cavity, green = gross tumor). Three overlapping dose calculation matrices are shown by green boxes (alternating dotted and solid lines). Radiochromic film is visualized as a white streak and is localized by the red reticule. The 4 Gy isodose line (yellow contour) results from the 27 shots planned to the resection cavity target and does not represent the composite 4 Gy isodose line of the total 40-shot plan ( Figure 2A ).
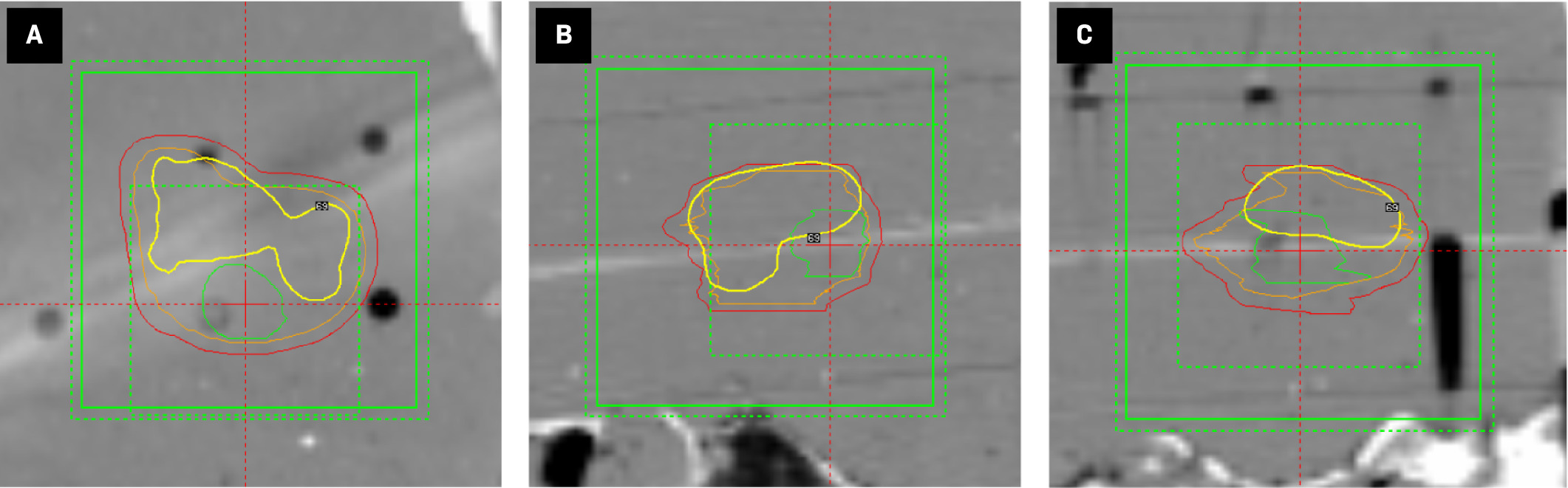
Axial view of simultaneous integrated boost Gamma Knife (Elekta) treatment plan for an anthropomorphic phantom ( A ) showing 3 target contours (red = 2-mm margin, orange = resection cavity, green = gross tumor) and isodose lines of 3 prescription doses (yellow contours: 3 Gy, 4 Gy, and 5 Gy). Radiochromic film of the delivered treatment plan calibrated for absolute dosimetry ( B ) with isodose lines of 3 prescription doses (red = 3 Gy, yellow = 4 Gy, green = 5 Gy) and color-scale legend of absolute dose (Gy). Gamma index analyses of radiochromic film at 1%/1-mm ( C ) and 2%/2-mm ( D ) tolerances with color-scale legend showing gamma index values (values <1 represent a passing score for a given point).
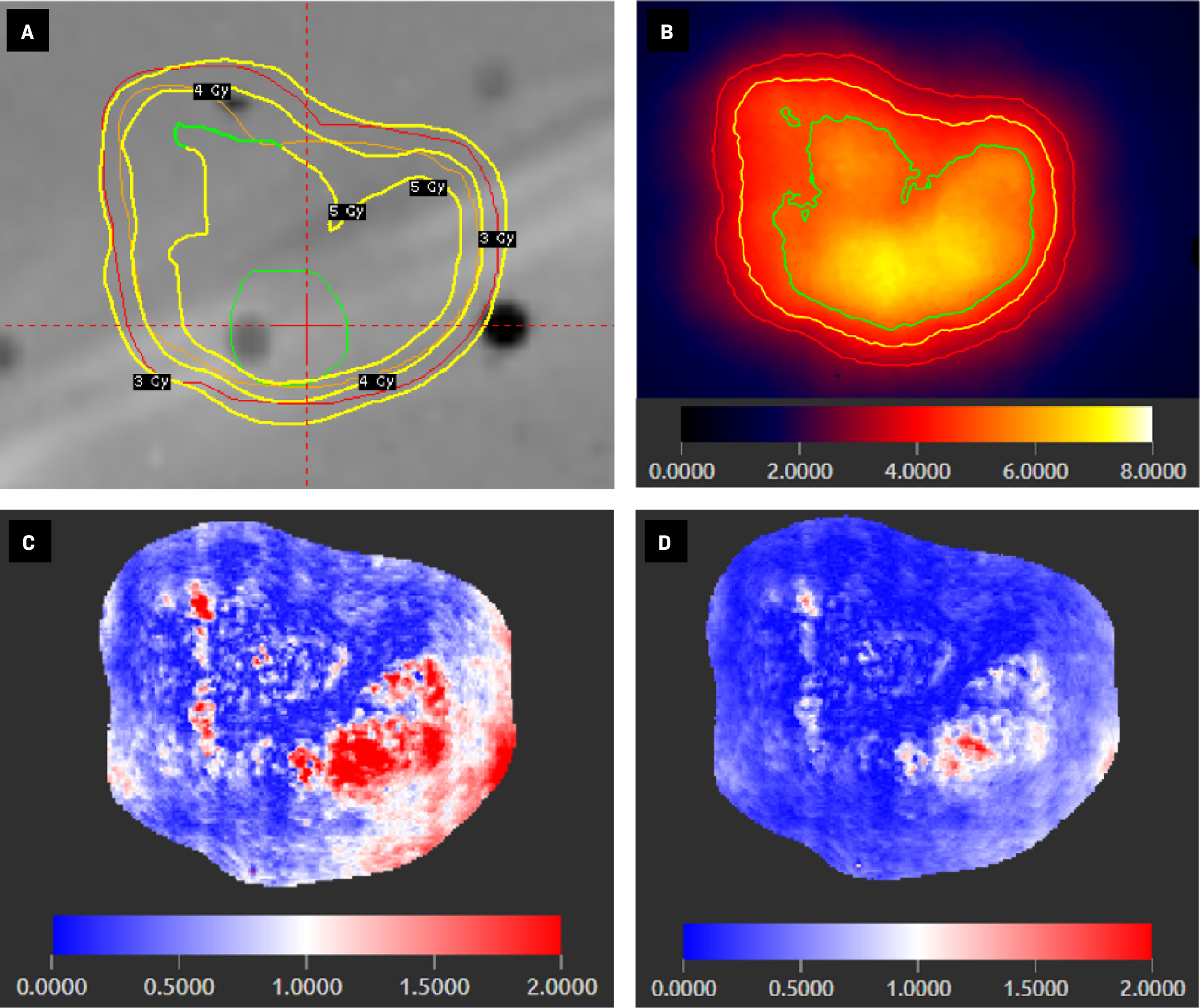
Results
Using LD and BOT penalty settings of 0.60 and 0.90, respectively, an 18-minute treatment plan with 40 shots was created after 221 seconds of optimization. The plan included 7 shots to the 2-mm margin (2.01 min of BOT), 27 to the resection cavity (12.63 min), and 6 to gross tumor (3.14 min) ( Figure 3 ). Prescription isodose lines were 3 Gy at 55% (2-mm expansion), 4 Gy at 69% (resection cavity), and 5 Gy at 75% (gross tumor) ( Figure 2A ). All target volumes had greater than or equal to 99% prescription dose coverage and the maximum dose was 7.0 Gy. Mean doses were 4.8 Gy (2-mm expansion), 5.2 Gy (resection cavity), and 6.1 Gy (gross tumor). PCI values were 0.79 (2-mm expansion), 0.74 (resection cavity), and 0.15 (gross tumor). GI was only available for the resection cavity (value = 2.5). On absolute dosimetry with Gafchromic film, the measured maximum dose was 7.112 Gy, 1.6% higher than planned. Gamma index pass rate, mean, and median values were 77%, 0.68, and 0.54 at 1%/1-mm tolerance, 85%, 0.58, and 0.49 at 2%/1-mm tolerance, and 97%, 0.34, and 0.28 at 2%/2-mm tolerance ( Figure 2 ). An average of 17,883 points were evaluated per gamma index analysis.
Axial, coronal, and sagittal views (arranged from left to right) of Gamma Knife (Elekta) shot position for the gross tumor ( A ), resection cavity ( B ), and 2-mm expansion ( C ) target volumes. The yellow contour represents the prescription isodose line for each target (2-mm expansion = 3 Gy, resection cavity = 4 Gy, gross tumor = 5 Gy) resulting from the contribution of shots planned to the given target, as opposed to the total 40-shot plan ( Figure 2A ).
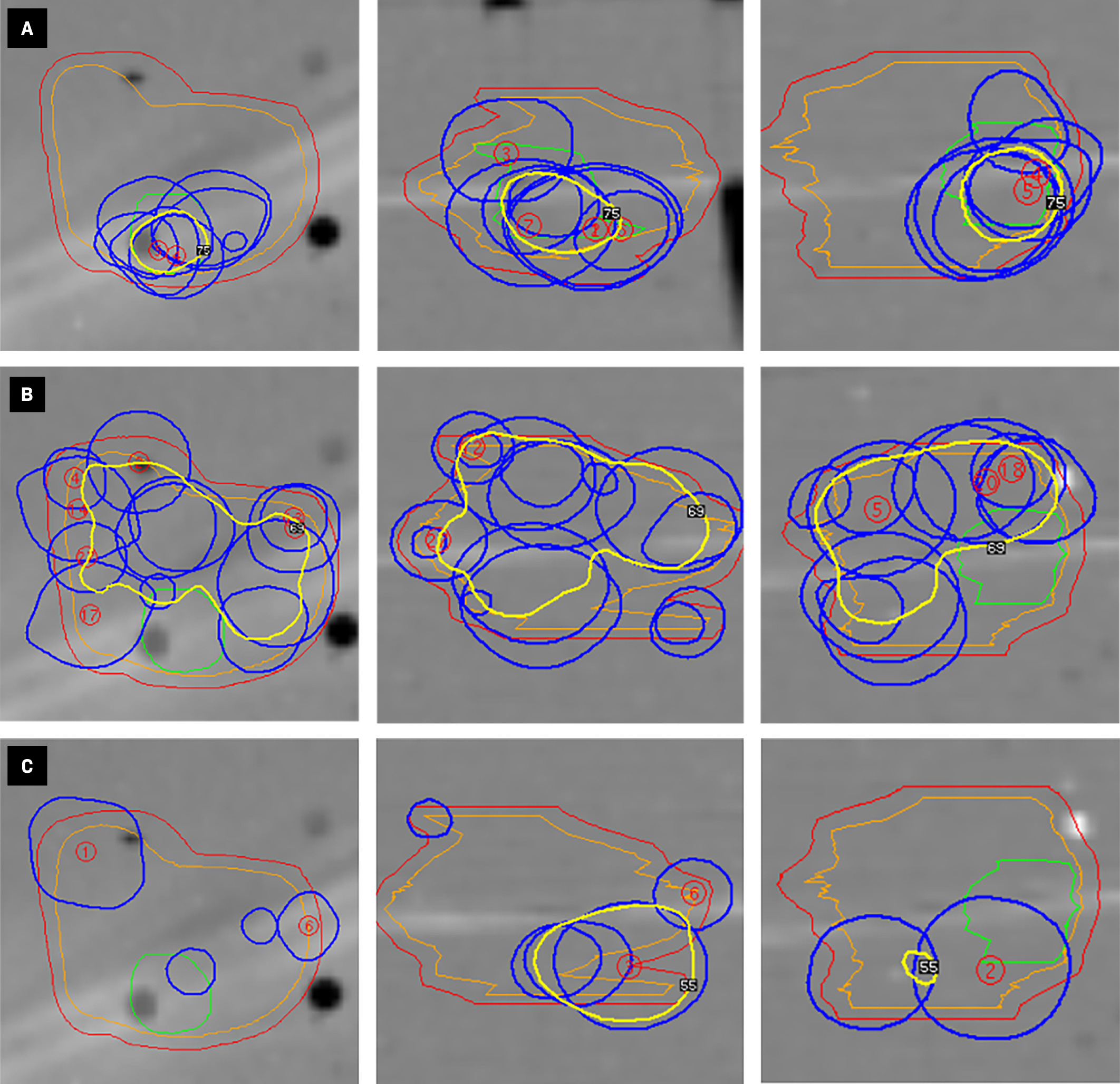
Discussion
We demonstrated a method for conducting an SIB using the GK Lightning optimizer and validated dose delivery within acceptable clinical thresholds.8, 19 This method allows for concurrent optimization of multiple nested dose volumes, provides dosimetric statistics for each volume, and does not require significant user experience. Additionally, this technique can be applied to concurrently treat multiple brain lesions with an SIB. However, as the GammaPlan user interface is not optimized for SIB planning, this method may be prone to interpretive error. This is exemplified by the nonintuitive interpretation of individual isodose lines when looking at a single target volume ( Figures 1 , 3 ), as well as the lack of a color gradient for prescription isodose lines within a composite treatment plan ( Figure 2A ). Additionally, since shots are delivered sequentially on a target-by-target basis ( Figure 3 ), and the total dose delivered to a given voxel is the summation of dose sequentially delivered to multiple targets ( Figure 2A ), this method may result in heterogeneous dose rate variability with an undetermined radiobiological significance and be more sensitive to intrafraction positioning errors.20
Clinically, our demonstration used the example of recurrent or residual tumor at the time of adjuvant radiation therapy for BM. This work does not address the optimal number of nested treatment volumes, the necessity of treating a radial margin, nor does this work define the most appropriate dose levels or fractionation pattern.21 - 24 For the treatment of BM, it is not well defined if an SIB improves outcomes or reduces toxicity, but this SIB method allows for standardization of treatment technique in future clinical trials designed to improve the therapeutic ratio of adjuvant stereotactic radiation therapy.25 The application of this SIB technique is not limited to adjuvant BM therapy as it could be used to deliver internal boosts to primary brain tumors via lattice radiation therapy.26, 27 Prospective work is needed to optimize the clinical application of this technique to elucidate appropriate clinical indications and dose levels.
Conclusions
We successfully created an SIB plan with the GK Lightning optimizer and verified dose delivery within clinically acceptable tolerances. Future work is needed to determine optimal dose levels for use in clinical practice and what disease entities may benefit from an intracranial SIB.
References
Citation
Barbour AB, Gates E, Ford E, Phillips M, Halasz LM. Dose Painting With the Gamma Knife Lightning Dose Optimizer: Technical Description and Validation of Dose Delivery. Appl Radiat Oncol. 2024;(2):15 - 20.
doi:10.37549/ARO-D-24-00012
June 1, 2024