Gastric Fistula After MR-Guided Stereotactic Body Radiation Therapy for Hepatocellular Carcinoma
Case Summary
The patient is a 68-year-old man with a history of hepatitis C, hypertension, hyperlipidemia, and an initial diagnosis of American Joint Committee on Cancer stage 2 (cT2N0M0), Child-Pugh class A, multifocal hepatocellular carcinoma (HCC). Eight years prior, he received multiple courses of chemoembolization. A surveillance follow-up CT scan recently demonstrated a solitary, active tumor in segment 2/3 abutting the stomach. Following his presentation at a multidisciplinary tumor board, he was referred for possible stereotactic body radiation therapy (SBRT). The patient reported feeling well overall, although he did endorse mild, intermittent episodes of nonradiating abdominal pain. Upon physical examination, there was no evidence of jaundice, ascites, or abdominal tenderness. His recent lab values included an international normalized ratio (INR) of 1.05, albumin of 4.2 g/dL, bilirubin of 0.8 mg/dL, and an AFP of 21.2 ng/mL. INR was measured to evaluate blood clotting and anticoagulation, and AFP was measured as a liver tumor marker.
Imaging Findings
His most recent CT scan of the abdomen demonstrated a single site of active disease within segments 2 and 3 of the liver measuring up to 7 cm with an exophytic component causing abutment of the stomach (
Patient’s initial CT scan showing active disease within segments 2 and 3 of the liver measuring up to 7 cm.
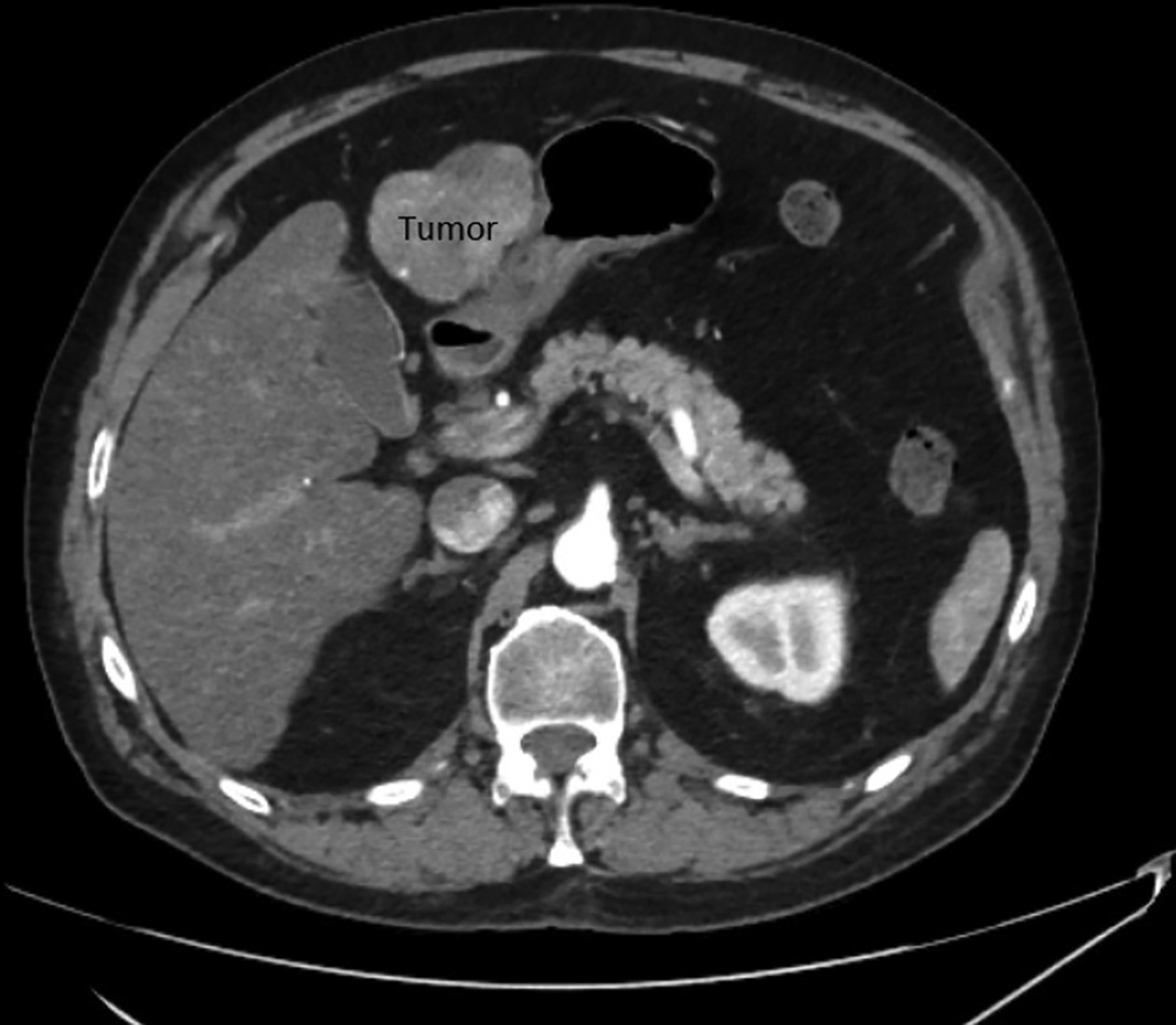
Isodose lines for MR-guided stereotactic body radiation therapy (MRgSBRT) treatment (A-E). (L= left, R = right, A in the bottom left corners = anterior)
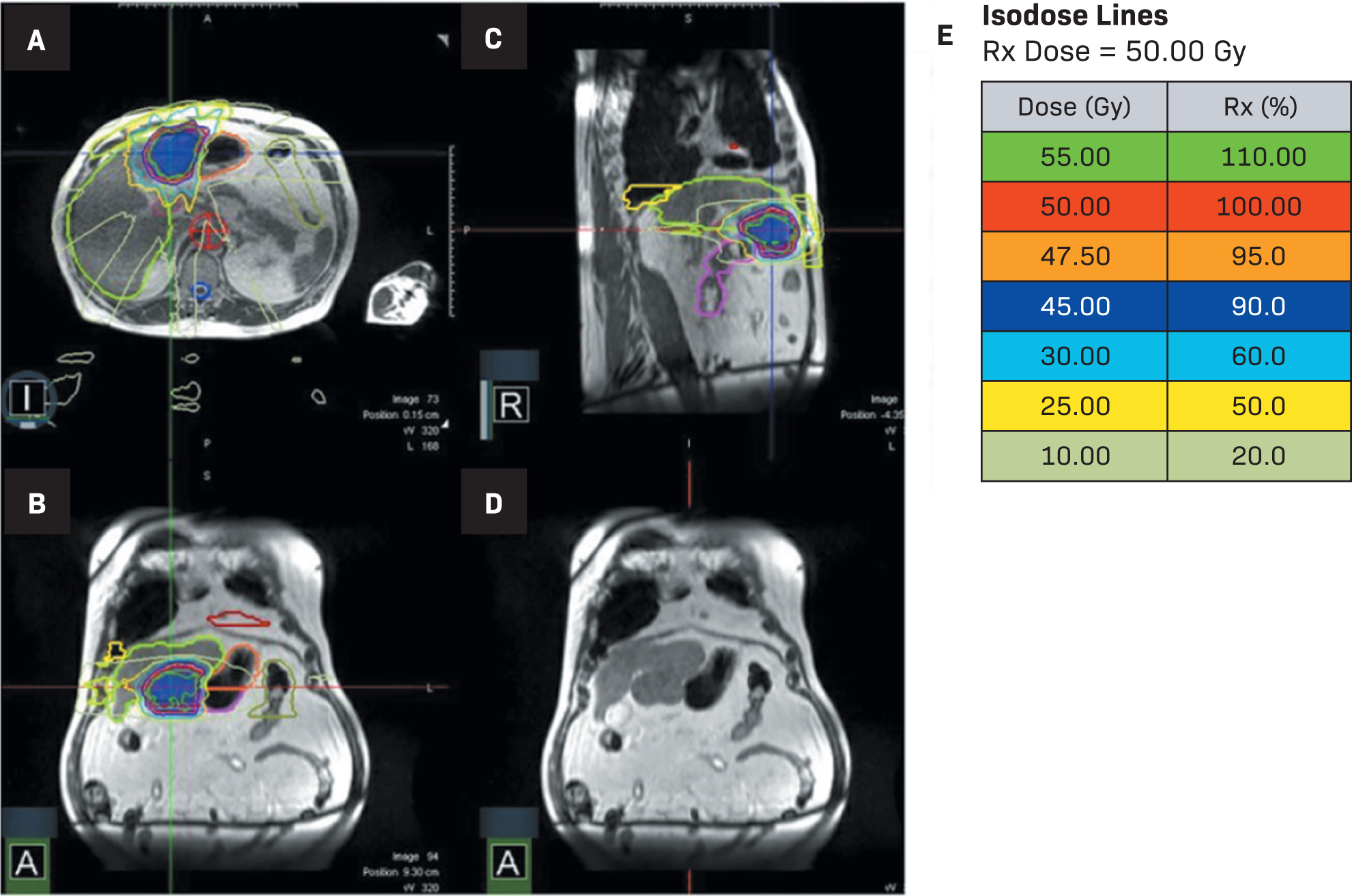
Dosimetric Constraints
GOALS | ACHIEVED | ||
---|---|---|---|
BR-001 | RTOG 1112 | PATIENT | |
Stomach | V35 < .5 cc | V30 < .5 cc | V35 at .01 cc |
Duodenum | V30 < .5 cc | V30 < .5 cc | V30 at .14 cc |
Bowel | V40 < .03 cc | V30 < .5 cc | V30 at .00 cc |
Abbreviation: RTOG, Radiation Therapy Oncology Group.
At the 3-month follow-up, the patient endorsed intermittent episodes of nausea without vomiting and mild, intermittent episodes of nonradiating abdominal pain that had remained stable since before treatment. The patient denied any fever or chills, and there was no evidence of ascites or abdominal tenderness. Lab work revealed a decrease in AFP to 10.6 ng/mL. A triple-phase CT scan of the abdomen showed a slight decrease in size of the treated left liver mass measuring 4.6 × 5.3 cm with gas and fluid components and communication to the lumen of the stomach.
Diagnosis
The patient in this case completed adaptive MRgSBRT for 50 Gy in 5 fractions (
The results of the triple-phase CT at the 3-month follow-up were consistent with gastric wall invasion and fistulization (
A 3-mo follow-up CT scan showing axial (A) and coronal views (B). A 6-mo follow-up CT scan showing axial (C) and coronal views (D).
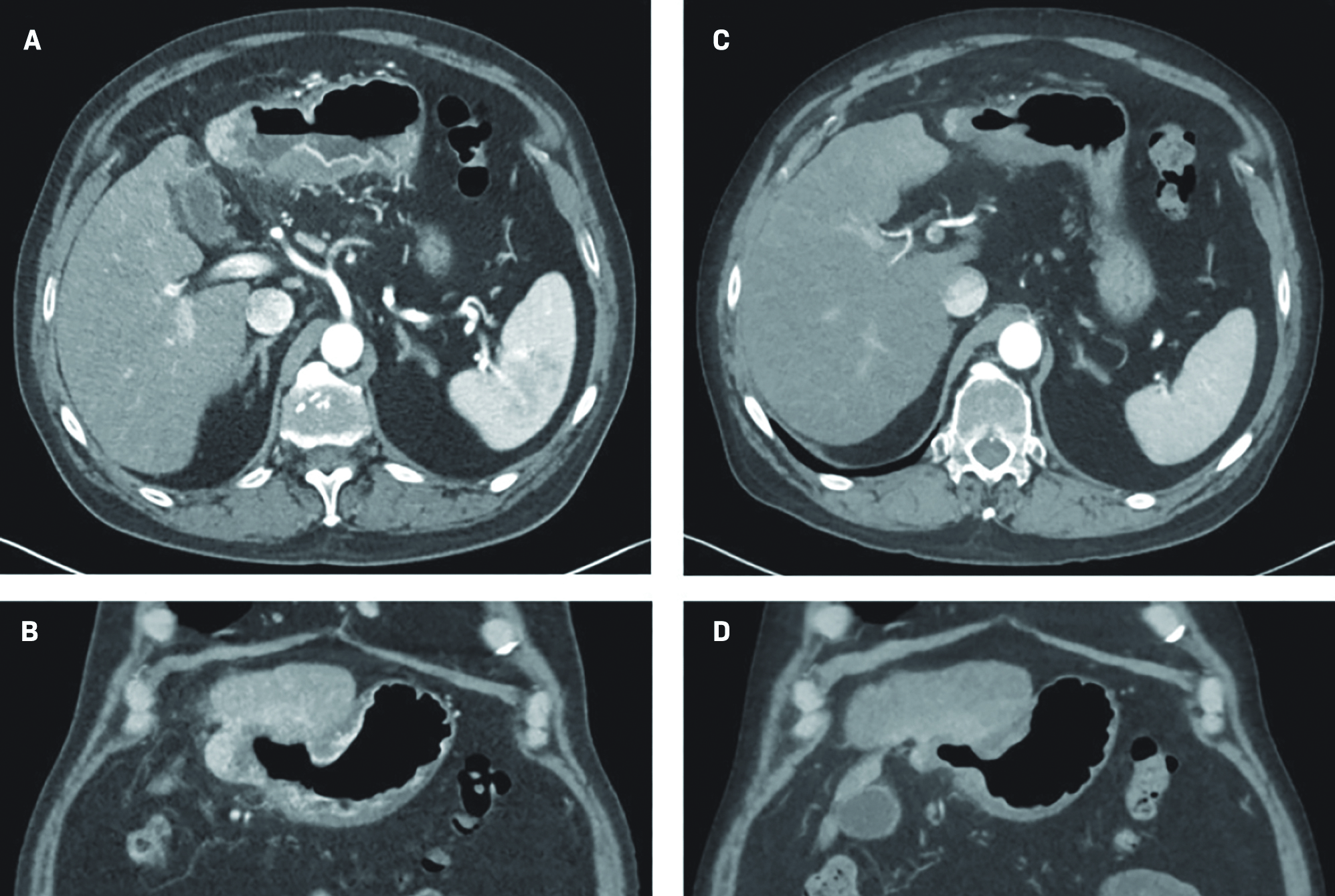
At the 6-month follow-up, the patient reported feeling well, the abdominal examination was without abnormality, and the lab work revealed an alpha-fetoprotein (AFP) value of 16.1 ng/mL. A repeat triple-phase CT of the abdomen demonstrated continual decrease in size of the treated segment 2/3 left hepatic lobe mass, with an internal air-fluid level, and stable fistulization to the stomach that measured 2.1 × 3.4 cm (
As the fistula had not changed significantly upon imaging and he remained asymptomatic, the patient was advised to continue PPI therapy and seek medical care should symptoms. He was referred to interventional radiology for consideration of percutaneous ablation for his progressive hepatic segment 4A lesion given the favorable location and size.
Discussion
Hepatocellular carcinoma is the most common primary cancer of the liver.3 The preferred mode of treatment is transplant or resection with locoregional therapies such as ablation and external beam radiation therapy (EBRT) as bridging treatments.3 Only 10% to 30% of patients at diagnosis are surgically eligible.4 In nonsurgical cases, locoregional therapy is used as a primary treatment. These established local treatments include interventional radiology ablation, arterially directed therapies, and EBRT.3,5,6
Stereotactic body radiation therapy is a form of EBRT that delivers precise high doses of radiation to a tumor—typically in the dose range of 30-50 Gy in 3-5 fractions.3 SBRT for HCC has potential benefits that include a decreased amount of normal tissue irradiation, a shorter overall treatment, and high 1-, 2-, and 3-year control rates of 87% to 93%, 74% to 89%, and 86%, respectively.7,8 Although prospective studies have reported high rates of local control and low rates of morbidity, until recently there was no reported increase in survival.9 In the recently reported RTOG 1112 trial, there is now a reported survival advantage of SBRT plus sorafenib (median overall survival, OS, = 15.8 mo) compared with SBRT alone (median OS = 12.3 mo).9 With the recent evidence supporting the role of immunotherapy (IO) in HCC, there is now also interest in exploring SBRT in combination with IO, but currently, there are little data regarding outcomes.10-13
Due to the proximity of GI organs-at-risk (OARs), there is an increased risk for toxicity after SBRT to the liver. Late reactions and toxicities from SBRT occur 3 or more months after the completion of radiation therapy and include gastritis, ulceration, perforation, and significant GI bleeding.14 Fistulation is a rare secondary consequence of ulceration that occurs at a rate of >5%. In a meta-analysis involving 1950 HCC patients treated with SBRT, grade 3 or higher hepatic and GI toxicities were 4.7% and 3.9%, respectively.5 Within GI toxicity, 10.5% of patients experienced a grade 3 or higher toxicity, including grade 4 gastric ulcer perforation in 4.3% of patients.5 Tolerance doses for structures such as the esophagus, stomach (
New technologies that improve the precision of SBRT delivery, such as MRgSBRT, have allowed for a reduced risk of toxicity for abdominal SBRT.16-18 The MR linac produces superior soft-tissue contrast and imaging while enabling daily imaging with sufficient quality that allows for daily plan adjustments according to interfraction organ motion.17,19 The target volume and OARs are recontoured daily.5 The use of an onboard cine-MRI during treatment allows for direct visualization of tumor motion, ensuring accuracy of radiation delivery while minimizing irradiating nondiseased tissue.12 For tumors with respiratory motion, guided breath holds allow for maintenance of the tumor’s position within the boundary for treatment and optimized target positioning.17 Studies have shown that 66% of liver fractions have benefited from online adaptation and that the online-adaptive planning revealed unintended OAR constraint violations that would have occurred in nonadaptive fractions at a rate of 63%.19 MRgSBRT optimizes the dose targeting the tumor while minimizing normal tissue irradiation, thus potentially widening the therapeutic index.
Studies evaluating the extent of intrafractional and interfractional liver motion on conventional linear accelerators (linacs) before and after SBRT have reported small variation, with Case et al showing that 80% of patients had a maximum amplitude of motion < 3 mm in any direction.20 However, in this study they did not have the capability to study motion continuously during treatment and tried to keep the treatment time < 25 minutes. There is little literature on the effects of intrafractional movement during MRI-guided radiation treatment. In a study that explored the effects of inter- and intrafraction movement, benefits from plan adaptations were noted.21 The results showed that the intrafractional adaptation was especially useful for high-dose OAR sparing.21 It is important to note that small variations—such as differences in respiratory phases and contouring variations—may influence high-dose OAR sparing. At our institution, we do not routinely track possible intrafractional movement of OARs throughout the course of treatment; however, we do track the tumor and that is a strength of the technology. With the upgrades that came after this patient was treated, clinicians have the ability to track intrafraction movement of multiple structures (tumor, isodose lines, and OARs).
Due to our patient’s Child-Pugh class A status and the large tumor size, we opted for a plan of 50 Gy in 5 fractions to maximize local control given his only site of disease. The biologically equivalent dose of 50 Gy in 5 fractions is analogous to the 3-fraction approach used in Child-Pugh A; if the patient was Child-Pugh B, the chosen dose would be 40 Gy in 5 fractions.22 While recognizing different lower dosage options in the RTOG 1112 trial (27.5-50 Gy), the radiosensitizer sorafenib used could allow for lower-dose sensitivities.2 Constraints under RTOG 1112 were still met under the utilized dosing scheme for this case. The entire time to adapt and deliver the treatment exceeded 1 hour daily. Although his stomach was contoured at the beginning of treatment, there was no mechanism to ensure that his stomach volume remained constant throughout. It is possible that he had additional gastric filling during his prolonged daily treatment times, and that the change could have accounted for increased cumulative dose to this region. At our institution, we do not have the capacity to do routine post-treatment imaging. However, in cases such as this with disease immediately adjacent to normal GI mucosal structures, perhaps mid- and post-treatment imaging should be routine so that the cumulative dosimetry can be confirmed.
Conclusion
This case demonstrates that despite the adoption of technological advancements that improve SBRT, incidents of severe side effects, such as fistulation, can still occur. MRgSBRT provides an adaptive method of treatment that allows for real-time, optimized normal tissue visualization to provide highly conformal, high-dose isodose lines that strictly adhere to dose constraints. More studies are needed to determine the extent of intrafraction tumor/normal tissue movement for upper abdominal tumor sites with treatment times approaching 1 hour.
References
- Dawson LA, Zhu A, Knox J Radiation therapy oncology group (RTOG) 1112 randomized Phase III study… Radiation Therapy Oncology Group. undefined. 2012; undefined(undefined):undefined-undefined
- Steve Chmura MD, Winter KA, Robinson C Safety of stereotactic body radiotherapy for the treatment of multiple metastases. JAMA Oncol. 2021; undefined(undefined):undefined-undefined
- undefined undefined. Hepatobiliary Cancer.. undefined; undefined(undefined):undefined-undefined
- Hoffe SE, Finkelstein SE, Russell MS, Shridhar R Nonsurgical options for hepatocellular carcinoma: evolving role of external beam radiotherapy. Cancer Control. 2010; 2(17):100-110
- Kimura T, Fujiwara T, Kameoka T, Adachi Y, Kariya S The current role of stereotactic body radiation therapy (SBRT) in hepatocellular carcinoma (HCC). Cancers (Basel). 2022; 18(14):undefined-undefined
- Salem R, Lewandowski RJ, Mulcahy MF Radioembolization for hepatocellular carcinoma using yttrium-90 microspheres: a comprehensive report of long-term outcomes. Gastroenterology. 2010; 1(138):52-64
- Ohri N, Tomé WA, Méndez Romero A Local control after stereotactic body radiation therapy for liver tumors. Int J Radiat Oncol Biol Phys. 2021; 1(110):188-195
- Wahl DR, Stenmark MH, Tao Y Outcomes after stereotactic body radiotherapy or radiofrequency ablation for hepatocellular carcinoma. J Clin Oncol. 2016; 5(34):452-459
- Tse RV, Hawkins M, Lockwood G Phase I study of individualized stereotactic body radiotherapy for hepatocellular carcinoma and Intrahepatic cholangiocarcinoma. J Clin Oncol. 2008; 4(26):657-664
- Abou-Alfa GK, Chan SL, Kudo M Phase 3 randomized, open-label, multicenter study of tremelimumab (T) and durvalumab (D) as first-line therapy in patients (PTS) with unresectable hepatocellular carcinoma (uHCC): Himalaya. JCO. 2022; 4_suppl(40):379-undefined
- Galle PR, Finn RS, Qin S Patient-reported outcomes with atezolizumab plus bevacizumab versus sorafenib in patients with unresectable hepatocellular carcinoma (IMbrave150): an open-label, randomised, phase 3 trial. Lancet Oncol. 2021; 7(22):991-1001
- Oh D-Y, He AR, Qin S A phase 3 randomized, double-blind, placebo-controlled study of durvalumab in combination with Gemcitabine plus cisplatin (GemCis) in patients (PTS) with Advanced Biliary Tract Cancer (BTC): Topaz-1. JCO. 2022; 4_suppl(40):378-378
- Jiang J, Diaz DA, Nuguru SP, Mittra A, Manne A Stereotactic body radiation therapy (SBRT) plus immune checkpoint inhibitors (ICI) in hepatocellular carcinoma and cholangiocarcinoma. Cancers (Basel). 2022; 1(15):undefined-undefined
- McKay MJ, Foster R Pathobiology, irradiation dosimetric parameters and therapy of radiation-induced gastric damage: a narrative review. J Gastrointest Oncol. 2021; 6(12):3115-3122
- Park J, Park JW, Kang MK Current status of stereotactic body radiotherapy for the treatment of hepatocellular carcinoma. Yeungnam Univ J Med. 2019; 3(36):192-200
- Rosenberg SA, Henke LE, Shaverdian N A multi-institutional experience of MR-guided liver stereotactic body radiation therapy. Adv Radiat Oncol. 2019; 1(4):142-149
- Boldrini L, Romano A, Mariani S MRI-guided stereotactic radiation therapy for hepatocellular carcinoma: a feasible and safe innovative treatment approach. J Cancer Res Clin Oncol. 2021; 7(147):2057-2068
- Stanescu T, Shessel A, Carpino-Rocca C MRI-guided online adaptive stereotactic body radiation therapy of liver and pancreas tumors on an MR-LINAC system. Cancers (Basel). 2022; 3(14):undefined-undefined
- Henke L, Kashani R, Robinson C Phase I trial of stereotactic MR-guided online adaptive radiation therapy (SMART) for the treatment of oligometastatic or unresectable primary malignancies of the abdomen. Radiother Oncol. 2018; 3(126):519-526
- Case RB, Moseley DJ, Sonke JJ Interfraction and intrafraction changes in amplitude of breathing motion in stereotactic liver radiotherapy. Int J Radiat Oncol Biol Phys. 2010; 3(77):918-925
- Lagerwaard F, Bohoudi O, Tetar S Combined inter- and intrafractional plan adaptation using fraction partitioning in magnetic resonance-guided radiotherapy delivery. Cureus. 2018; 4(10):undefined-undefined
- Price TR, Perkins SM, Sandrasegaran K Evaluation of response after stereotactic body radiotherapy for hepatocellular carcinoma. Cancer. 2012; 12(118):3191-3198
Citation
Goodchild S, Mills MN, Palm RF, Hoffe SE, Frakes JM. Gastric Fistula After MR-Guided Stereotactic Body Radiation Therapy for Hepatocellular Carcinoma. Appl Radiat Oncol. 2023;(4):1-6.
doi:10.37549/ARO-D-23-00014
September 1, 2023