Case ReportLung/Thoracic Cancer
High-Field MR-Guided Radiation Therapy for Oligometastatic Central Lung Cancer: Current State and Future Opportunities
Images
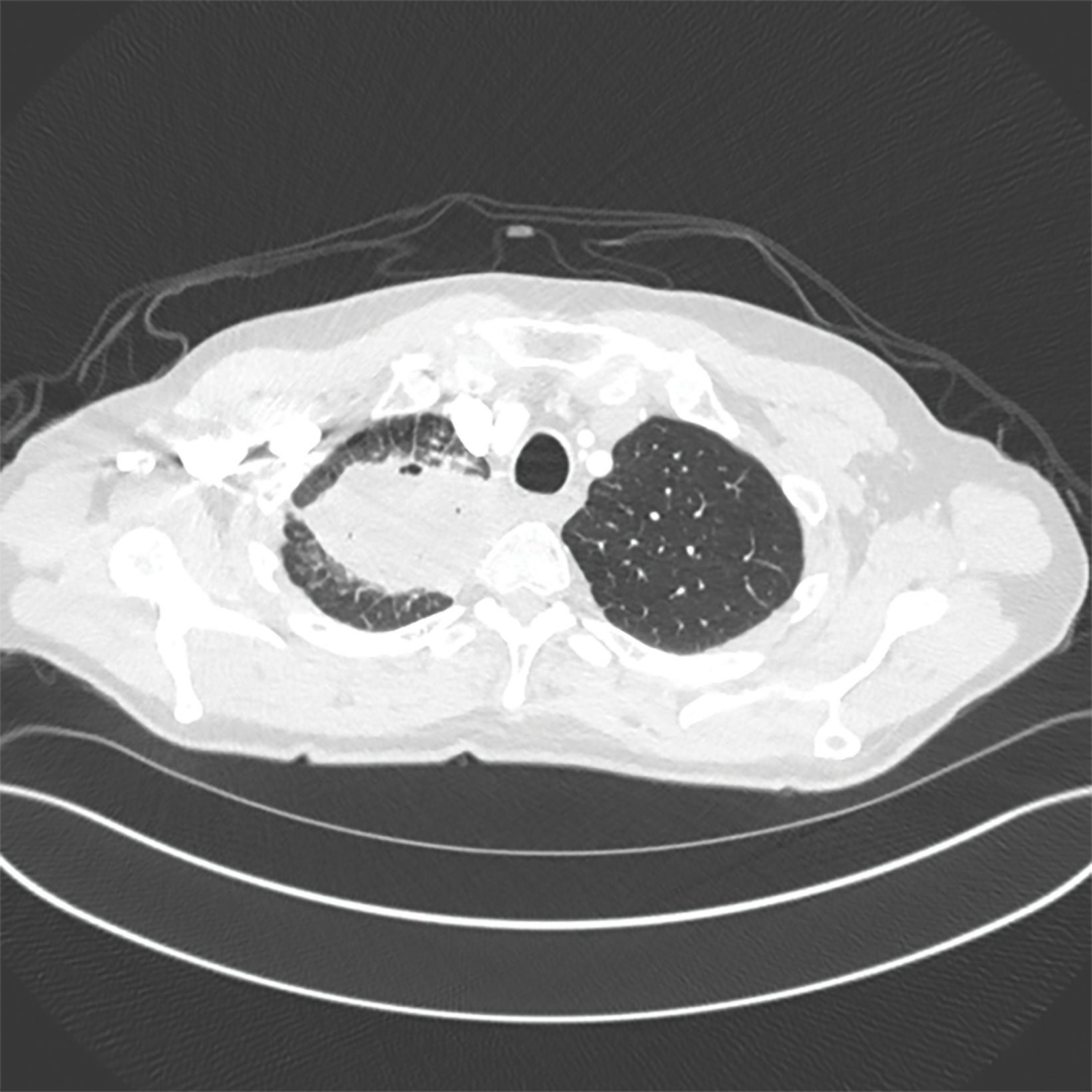
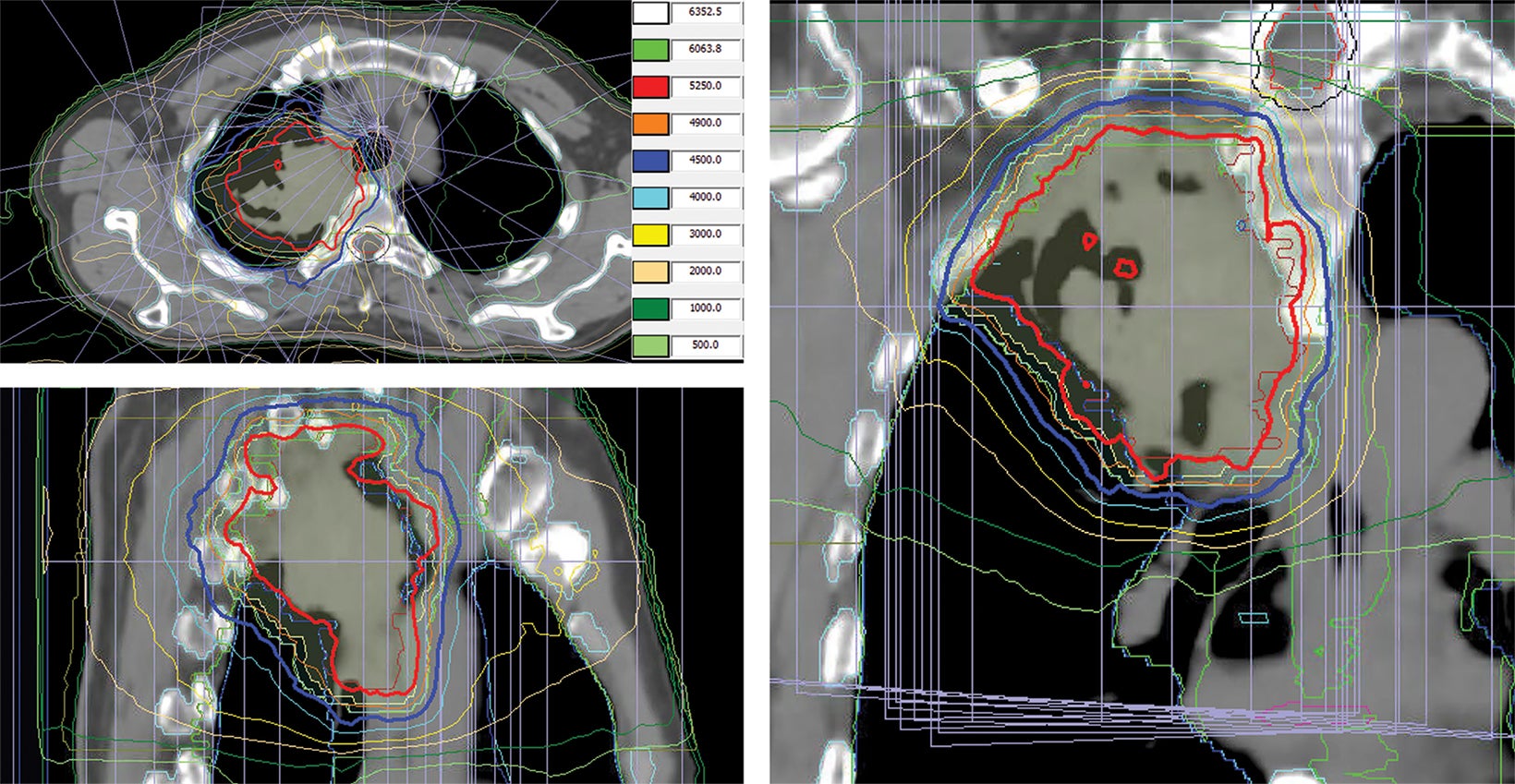
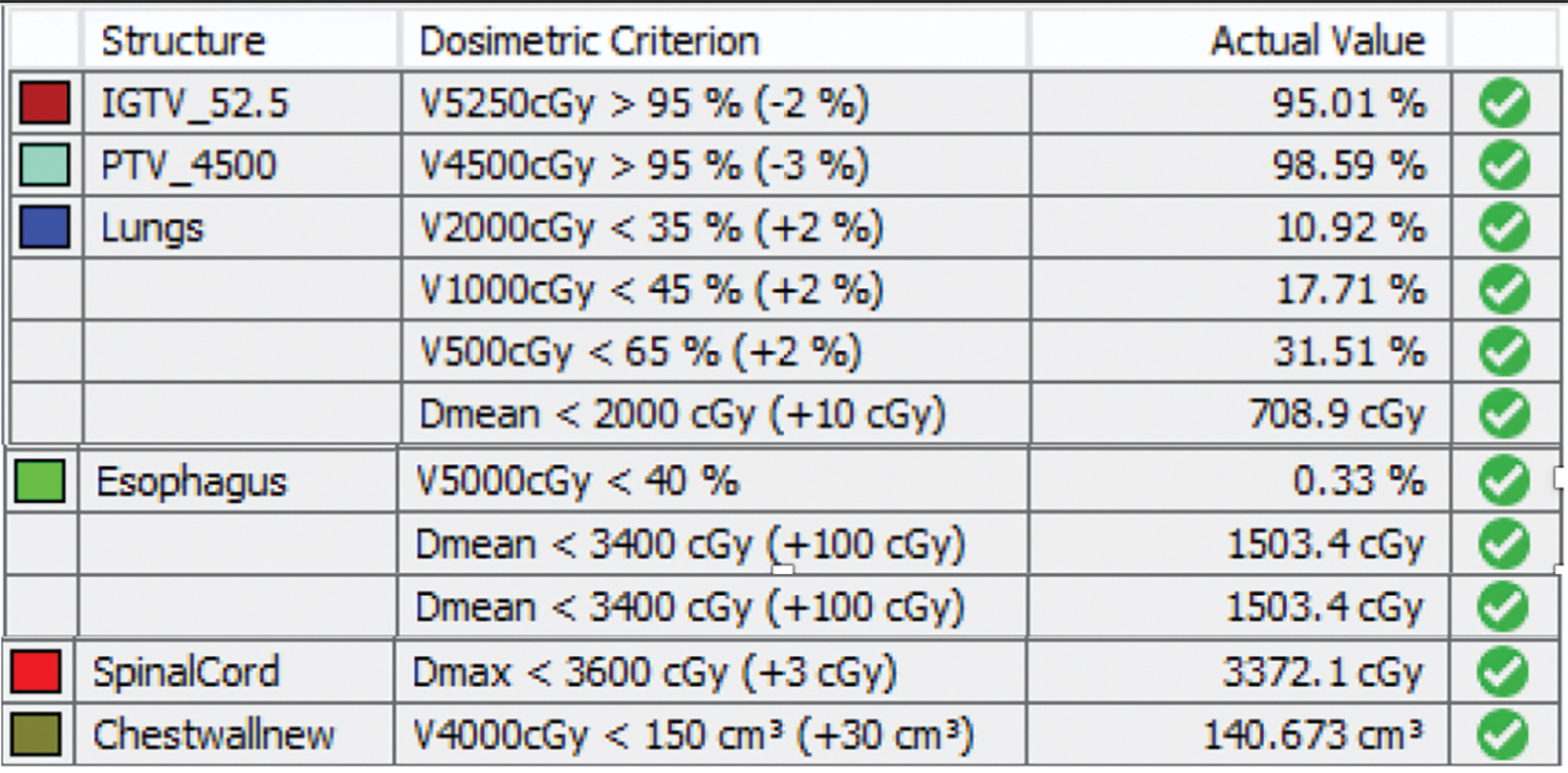
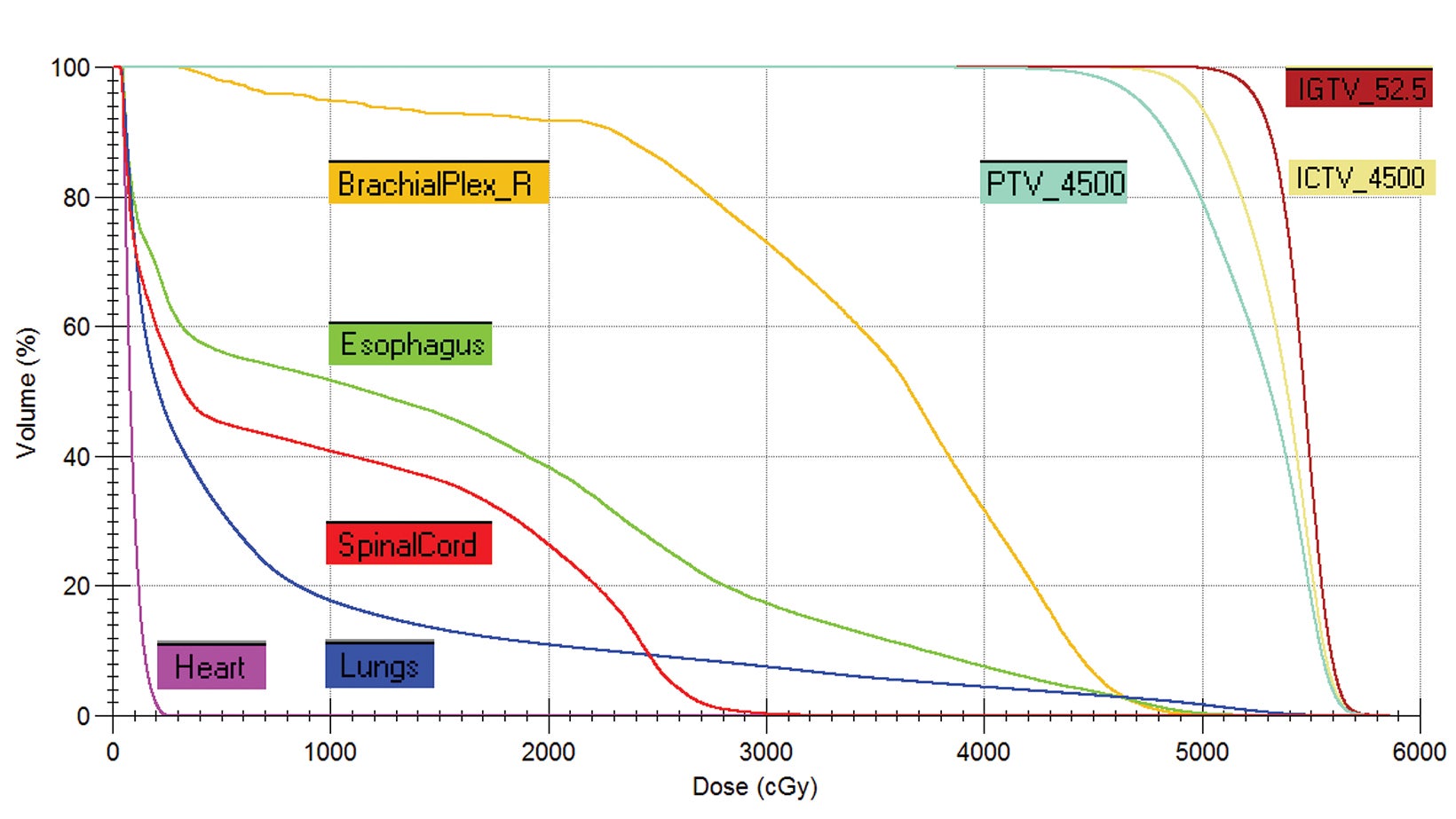
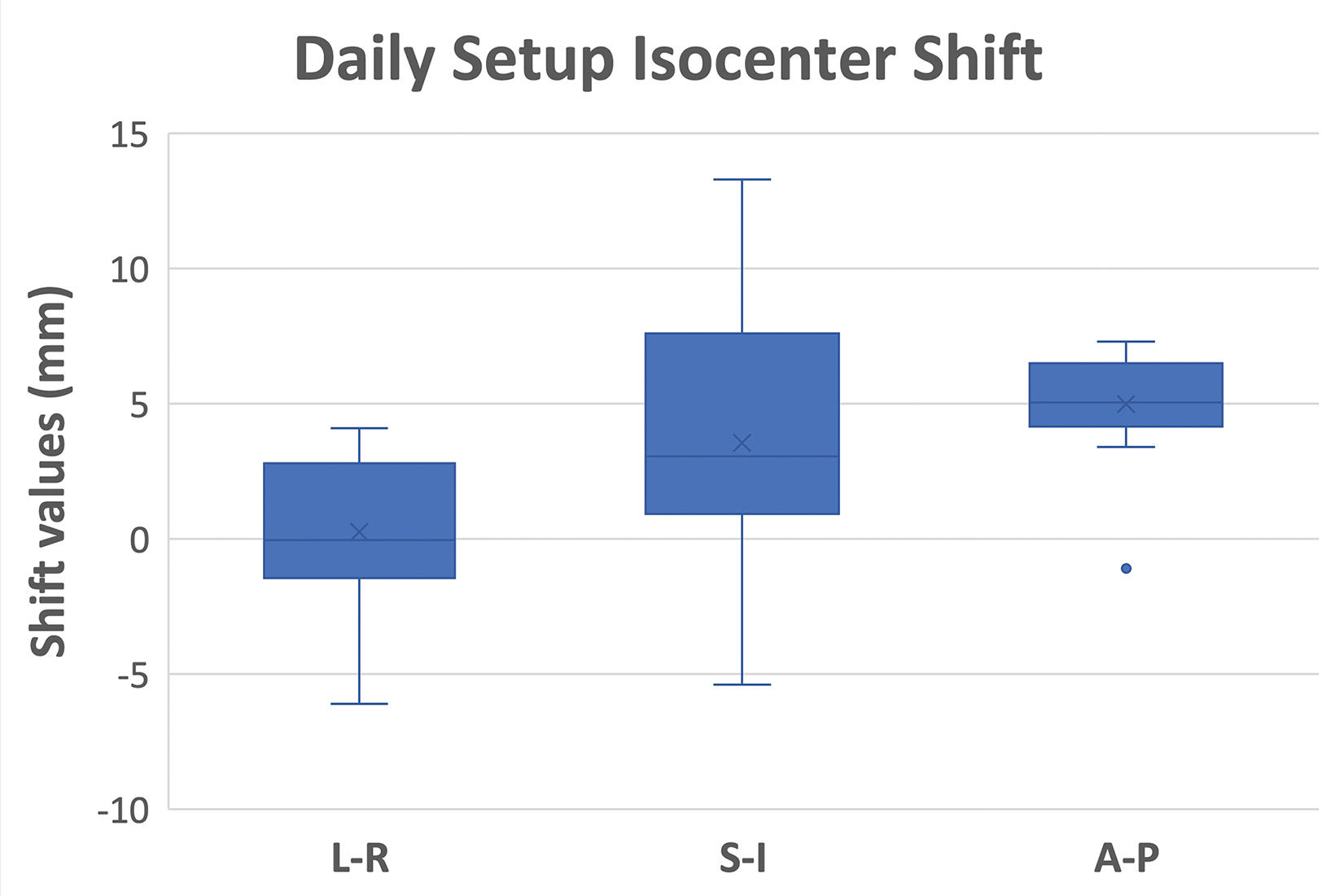
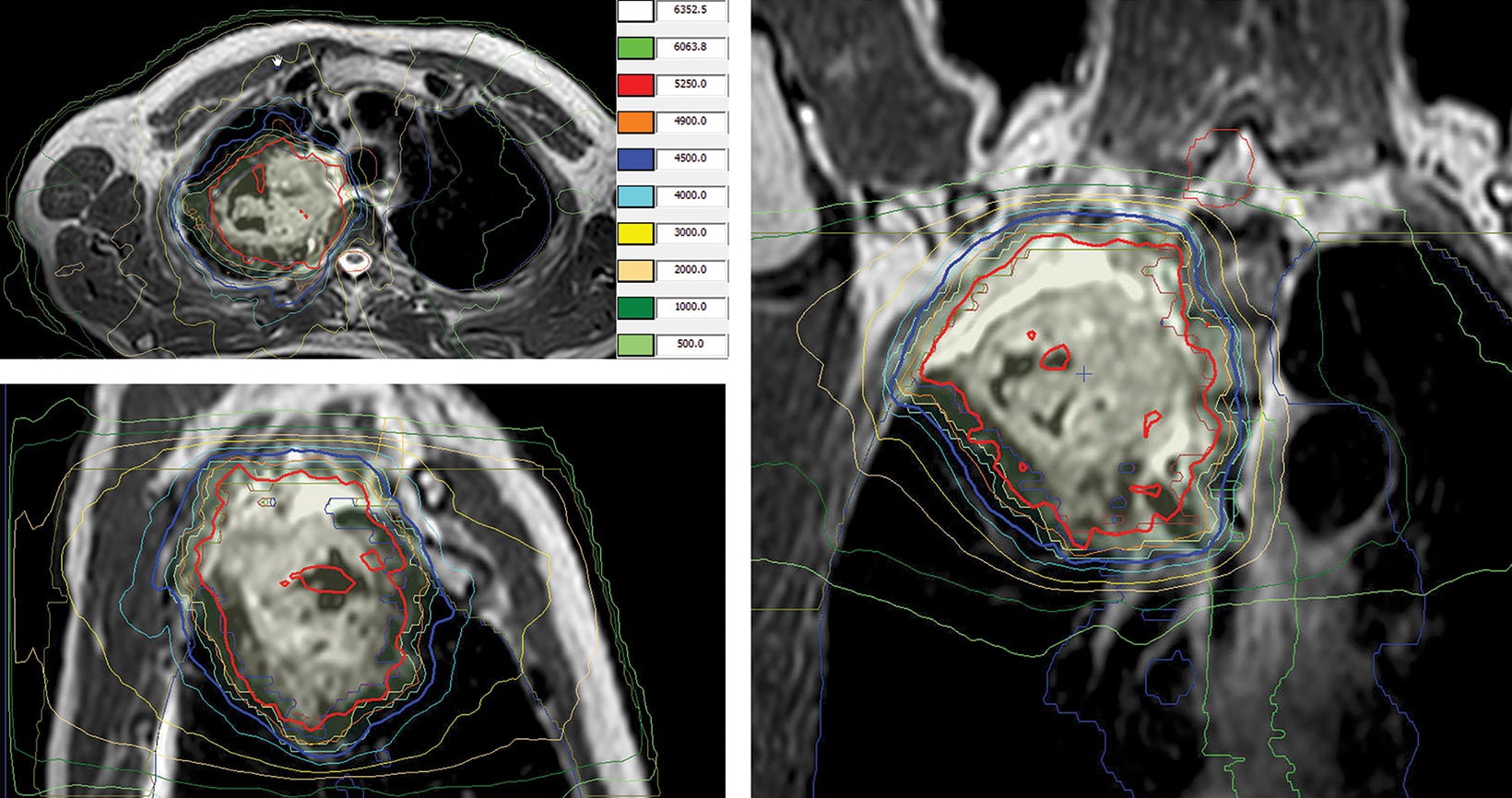
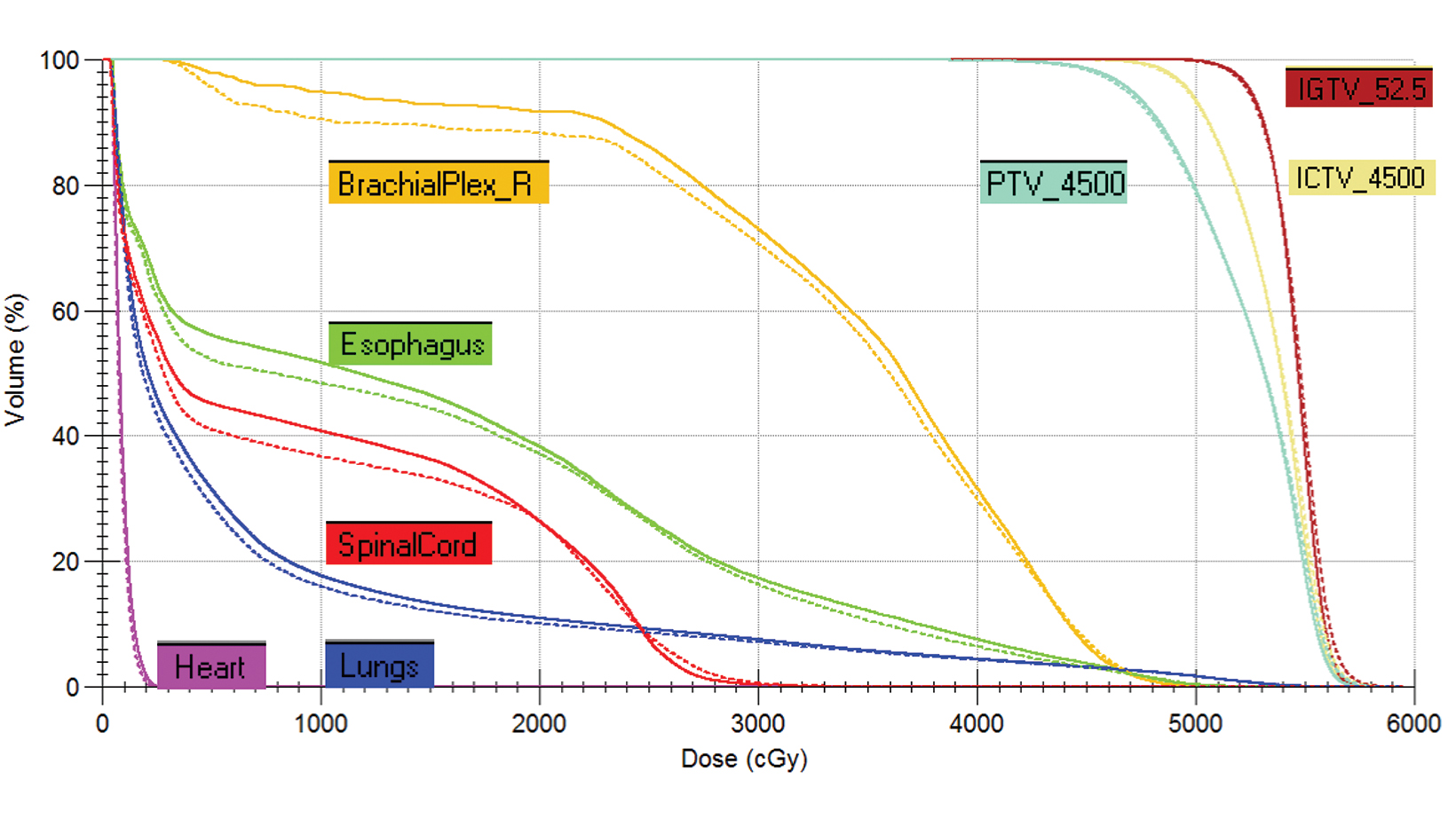
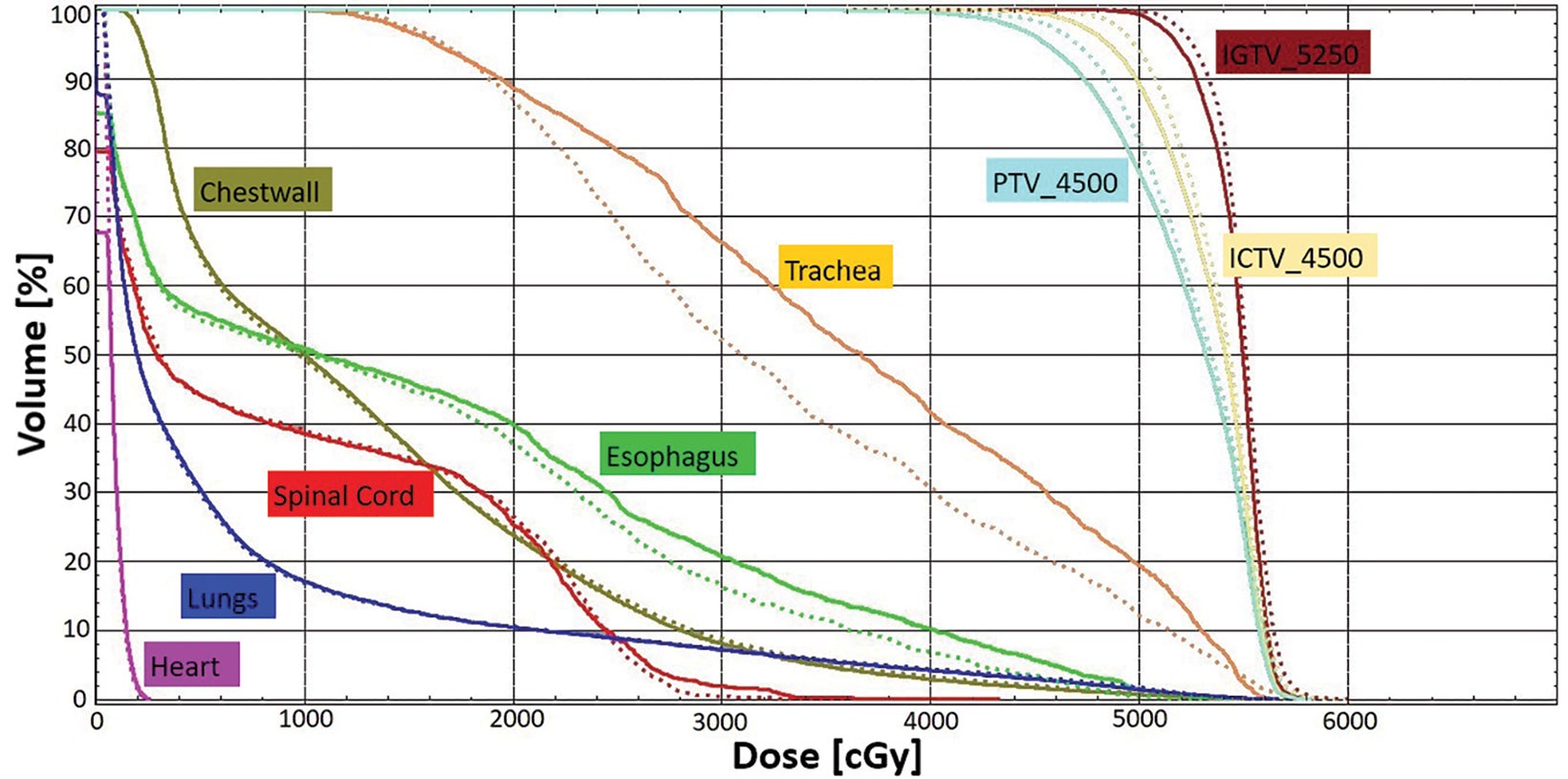
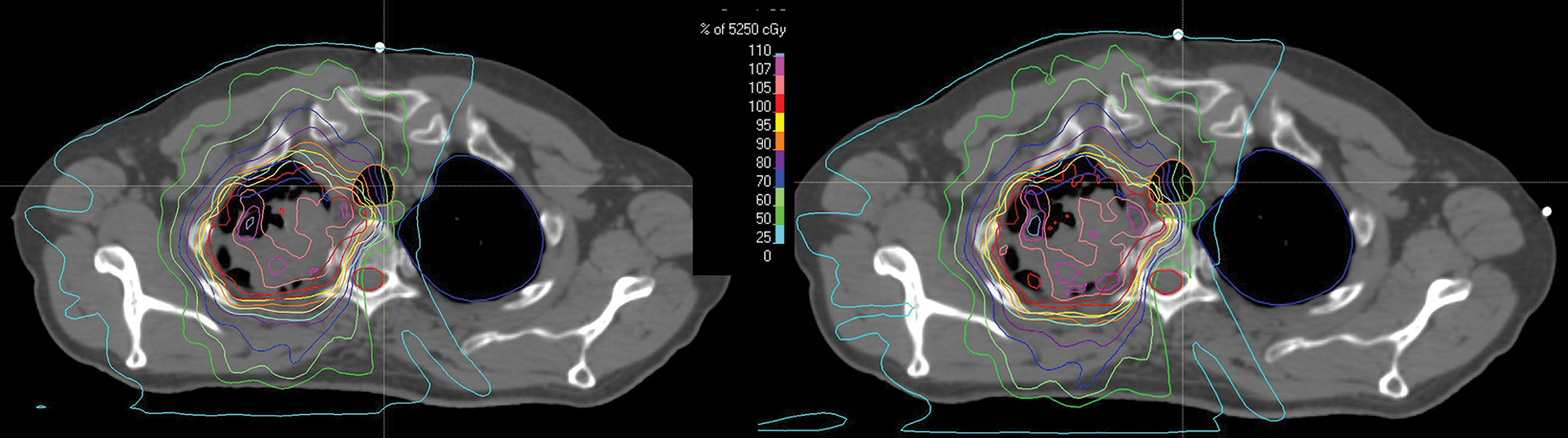
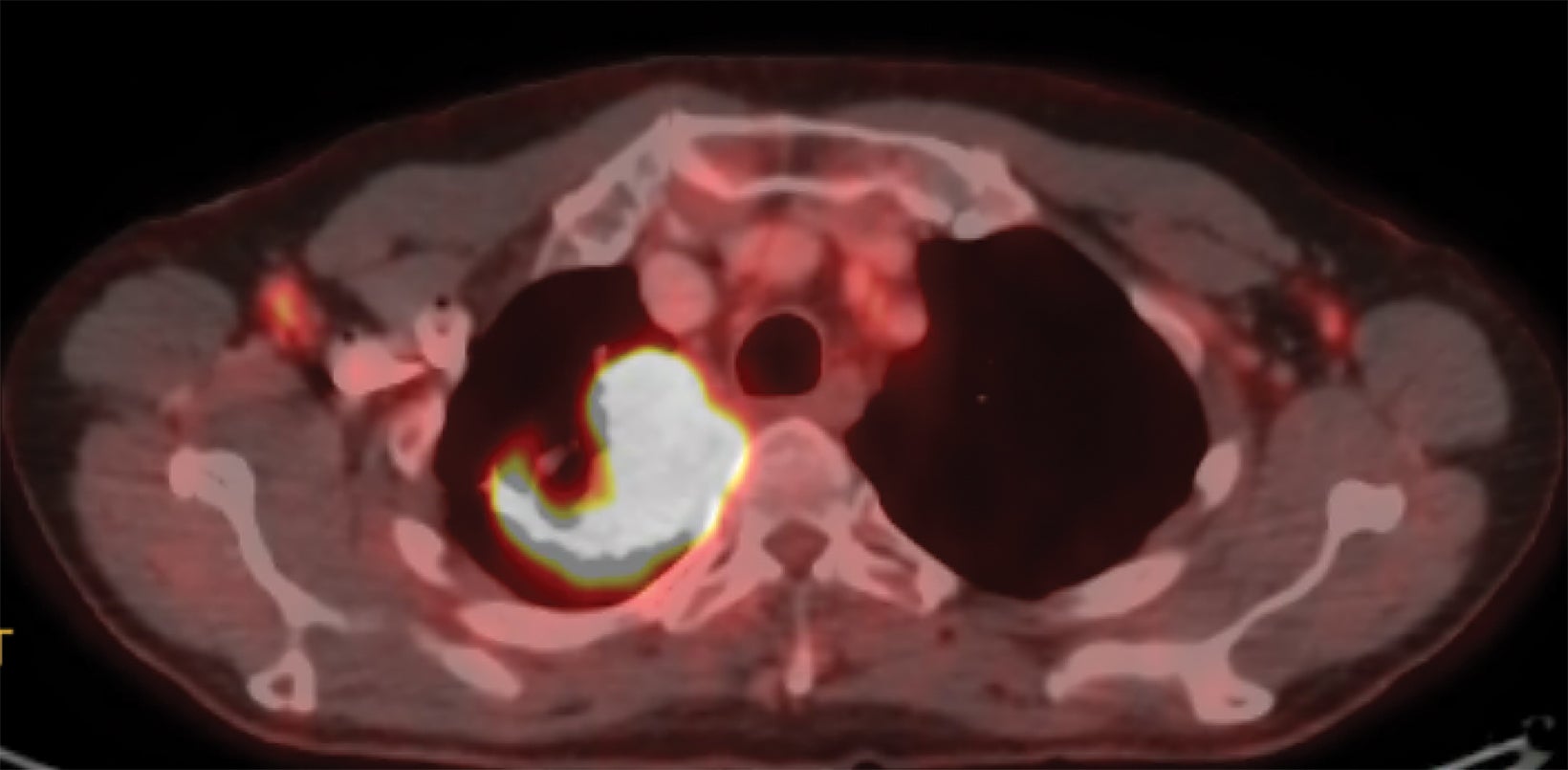
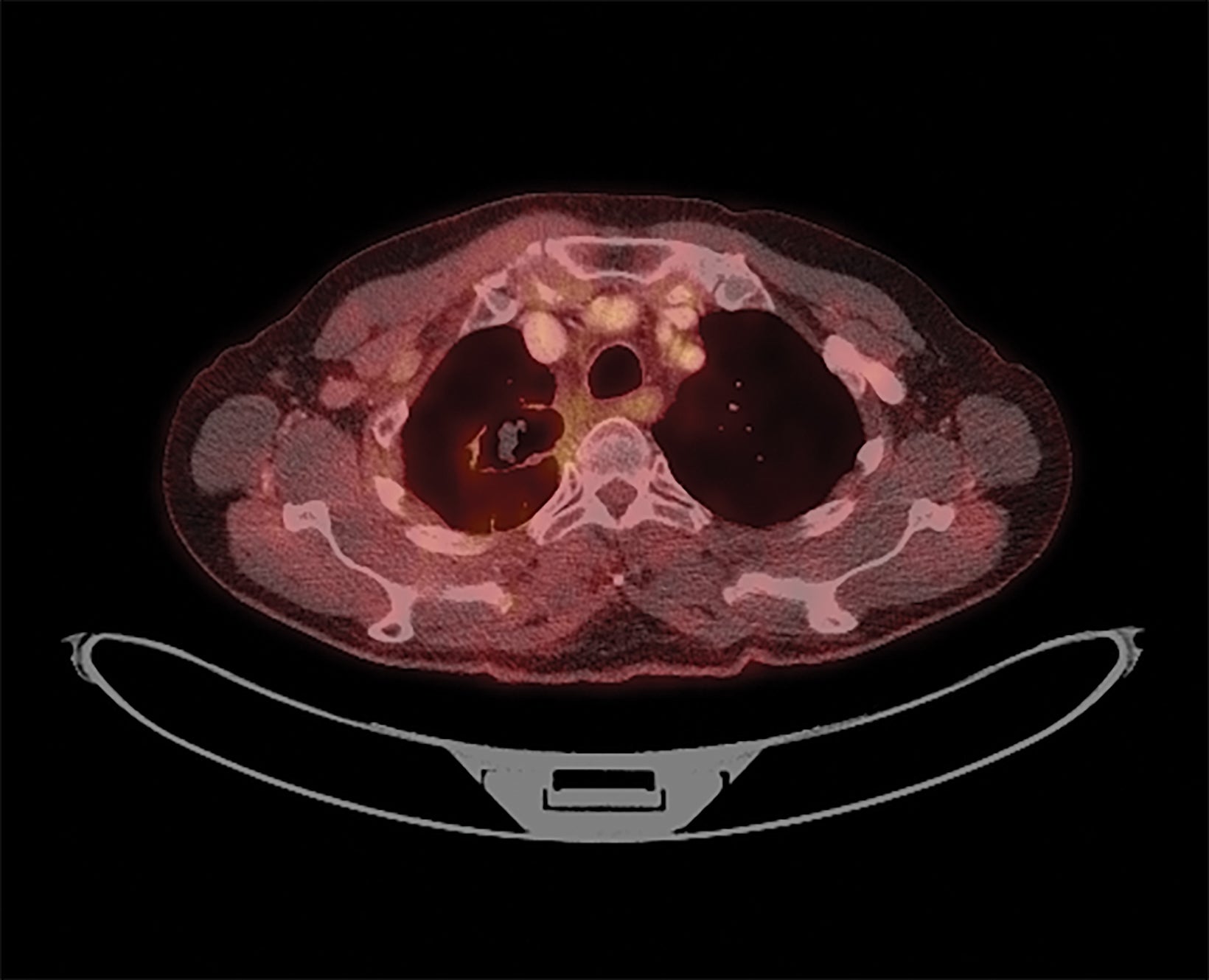
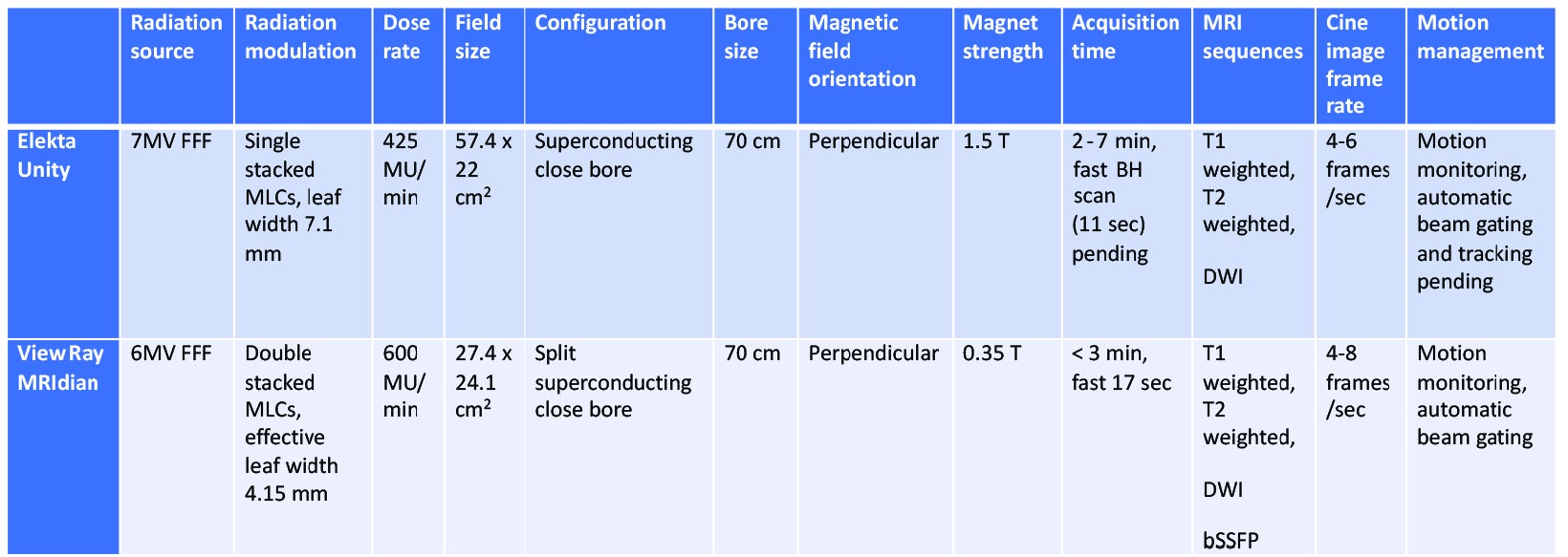
Case Summary
A 68-year-old never-smoker presented with a chronic cough for 1 year. A chest x-ray demonstrated a right upper lobe (RUL) mass-like consolidation and subsequent computed tomography (CT) of the chest confirmed a 7.8-×-4.6-cm RUL mass extending into the mediastinum (Figure 1). Needle biopsy revealed squamous cell carcinoma with PDL1 40% and MET exon 14 skipping mutation. Positron emission tomography (PET)/CT demonstrated oligometastatic disease with suspicious mediastinal lymphadenopathy and a 2.6-cm left iliac wing metastasis. Endobronchial ultrasound was negative for metastatic disease to nodal stations 7, 4, and 10R. MRI of the brain did not demonstrate concerning intracranial lesions.
The patient was started on carboplatin/paclitaxel/pembrolizumab for 1 cycle and developed a pruritic, full-body rash (grade 3) requiring steroids. He was subsequently enrolled on a clinical trial of tepotinib (MET inhibitor, NCT02864992). He received 6 cycles of tepotinib, but continued to have recurrent episodes of rash and scalp lesions that prompted frequent treatment interruptions. After receiving 4 months of tepotinib, he ultimately developed progressive disease in the thorax and tepotinib was discontinued. He was evaluated in the thoracic radiation oncology clinic and the recommendation was made for local consolidation therapy with concurrent chemoradiation. He received 52.5 Gy/15 fx to the RUL primary, which is one of the most common regimens at our institution for consolidative thoracic therapy for oligometastatic disease, and was included on the non-small cell lung cancer (NSCLC) local consolidation therapy study by Gomez et al.1 He also underwent 27 Gy/3 fx to his left iliac wing metastasis.
Given the central location of his primary tumor and proximity to critical structures such as the trachea, his RUL primary was treated on a 1.5 Tesla MR linear accelerator (MR-linac).
Simulation included a CT scan on a Philips Brilliance 16-slice CT scanner followed by MR scans on the MR-Linac. He was immobilized with an MR-compatible Vac-Lok cushion. The lung 4D CT scan protocol was performed at CT simulation. Average 4D CT was reconstructed for treatment planning, with image resolution of 1 × 1 × 2.5 mm. The MR scans included both 3D T1- and T2-weighted sequences, with reconstructed image resolution of 1 × 1 × 1.2 mm.
Target contouring was performed based on a combination of 4D CT, PET, and MRI by taking into account the uncertainty from respiratory motion. The target volume included all gross disease as defined on CT, PET and MRI with a 5- to 7-mm clinical treatment volume (CTV) and 5-mm planning treatment volume (PTV). Organs at risk (OARs) were contoured on CT for lungs, heart, esophagus, spinal cord, brachial plexus, and chest wall.
style="padding-left: 6pt;text-indent: 9pt;text-align: left;">The reference plan was done on the average 4D CT in the Monaco treatment planning system (Elekta). A step-and-shoot intensity-modulated radiation therapy (IMRT) plan was developed for treatment with the Elekta Unity MR-linac with beam energy of 7 MV flattening filter free. Twelve beams were used, with beam angles of 30, 0, 340, 320, 300, 280, 260, 240, 220, 200, 170, and 150. The dose calculation uses a graphics processing unit (GPU)-based Monte Carlo algorithm with a variance reduction technique.2 The dose grid was set to 3 × 3 × 3 mm3 and the statistical uncertainty of the Monte Carlo algorithm is 1%. A total dose of 52.5 Gy was prescribed to cover at least 95% of the envelope of respiratory motion of gross tumor volume (GTV) in 15 fractions (Figure 2). The final reference plan has a total of 1100 monitor units and 98 segments. Achieved target and normal tissue doses are depicted in Figure 3.
The quality assurance (QA) for the pretreatment patient chart followed the same guidelines for conventional linac treatments, including a secondary MU verification using RadCalc (Lifeline Software Inc.) and IMRT QA measurement using ArcCheck MR phantom (Sun Nuclear Corporation).
For each treatment fraction, the patient was set up to the couch index location recorded at simulation. There is no external laser in the MR-linac treatment room. The patient setup relied on an internal sagittal laser and leveling marks on patient surface. A 3D T2 MRI scan was acquired with an acquisition time of 2 minutes. This MR scan was rigidly registered to the simulation CT scan of the reference plan, accounting for the isocenter shifts in left-right (LR), anterior-posterior (AP), and superior-inferior (SI) directions. Figure 4 shows the statistics of the shift values of all 15 fractions. An adaptive plan was then created based on an “adapt to position (ATP)” approach. Specifically, the plan isocenter defined on the reference CT plan was virtually “shifted” to a new location on the CT scan based on isocenter shifts determined by the MR/CT registration. The original reference plan was then re-optimized to ensure that target and normal tissue planning constraints were met or maintained (Figure 5). Once the adaptive plan was accepted by the attending physician, a secondary MU check of the adaptive plan was performed before the plan was transferred to the linac console for delivery. On average, treatments were 35 minutes from patient in room to treatment completion. After all fractions were delivered, we performed dose accumulation of daily adaptive plans to evaluate the dosimetric accuracy of the ATP approach, which is often considered suboptimal because daily anatomy difference was not accounted for during plan adaptation. Each ATP plan dose was scaled down to 1 fraction and added together to create a summed dose, which represented the planned delivered dose. At the same time, the fraction dose of each ATP plan was shifted to the corresponding MR image and deformed back to the reference CT image for accumulation by using an in-house deformable registration tool.3,4
The accumulated deformed dose approximated the actual delivered dose by accounting for daily anatomic variations. By comparing the accumulated deformed dose with the summed plan dose, target dose difference is less than 1% for gross tumor volume, clinical target volume, and planning target volumes (Figure 6). Most OARs have a small dose difference, except the trachea receiving 10% more dose (3.3 Gy) and esophagus receiving 7.2% more dose (1 Gy). Dose distribution was compared in Figure 7. This demonstrates the potential benefit for using a truly adaptive process accounting for daily anatomical variation, adapt to shape (ATS), in the future. This is particularly the case if planned doses were higher and delivered using a stereotactic ablative approach in the future for central and ultracentral tumors to reduce tracheal and esophageal doses.
The patient tolerated radiation therapy (RT) well with a grade 2 cough and esophagitis during treatment. After RT, he had a persistent mild cough and dyspnea on exertion not requiring steroids. After RT, he received 3 cycles of consolidative carboplatin and taxol. PET at 3 months demonstrated partial response of the treated lesions (Figure 8), but also revealed suspicious hypermetabolic right, anterior pleural/chest wall lesions. On follow-up, his chest wall metastases became symptomatic and he was also found to have mediastinal progression inferior to the prior RT field, and new right lung nodules. He underwent 2 months of capmatinib and palliative RT to his chest wall metastases (30 Gy/10 fx). Although his pain improved, he continued to progress and was subsequently enrolled on a trial of Tisotumab vedotin with partial response but developed Guillain-Barre requiring intravenous immunoglobulin. He subsequently was enrolled on a trial of a MDM2 inhibitor with Taxol, but ultimately progressed and received palliative radiation to his left femur (12 Gy/1 fx) and lumbar spine (24 Gy/1 fx). On the most recent follow- up approximately 1.5 years after initial RT, he had transitioned to hospice care.
Diagnosis
Squamous cell carcinoma, PDL1 positive (40%), MET exon 14 skipping mutation
Discussion
The term oligometastatic disease refers to a distinct clinical state involving 1 to 5 metastases that are amenable to local therapies.5 Between 25% and 50% of metastatic NSCLC patients initially present with oligometastatic disease.6
A growing body of evidence suggests that consolidating all known sites of oligometastases with RT may increase progression-free survival (PFS) and overall survival (OS). In a multi-institutional, phase II trial, 49 NSCLC patients with ≤ 3 metastases were randomized to standard-of-care systemic therapy ± local consolidation therapy (LCT).1 The study was closed early by the data safety and monitoring board after interim analysis demonstrated significant PFS benefit. After a median follow-up of 39 months, there was also an OS benefit of 41 vs 17 months in favor of the LCT arm. Another phase II trial, SABR-COMET,7 randomized 99 patients with ≤ 5 metastases to standard-of-care systemic therapy ± LCT. This study enrolled patients of various histologies, including 18% with NSCLC. After a median follow-up of 51 months, the 5-year OS in the LCT arm significantly improved (42% vs 17%). Although these studies and others have demonstrated the benefit and relative tolerability of LCT, there remain risks of consolidative RT. On SABR-COMET, 3 patients (4.5%) died as a result of treatment-related toxicity-2 cases of which were related to lung-directed RT.
In radiation oncology, central lung tumors are typically defined as lesions within 2 cm of the proximal bronchial tree and are associated with the highest rate of pulmonary RT complications. This is due to the proximity of tumor to critical structures including the trachea, esophagus, heart, and great vessels. The challenges of treating central lung tumors also derive from difficulty in delineating tumor from soft tissue with conventional CT imaging, which increases uncertainty of appropriately achieving OAR dose constraints. As a result, at our institution8 we generally recommend hypofractionated RT regimens as opposed to stereotactic body radiation (SBRT) for bulky central lung tumors as described in the aforementioned patient case. Given the promising results of definitive doses of consolidative RT for oligometastatic disease, the reduction of OAR doses while maintaining a high biologic effective dose (BED) would represent a meaningful advance in the treatment of central NSCLC.
MR-guided radiation (MRgRT) is a significant technological innovation integrating a linear accelerator and MRI machine. Several notable advantages of MRgRT potentially increase the therapeutic ratio of radiation and are most beneficial for tumors adjacent to multiple OARs, such as central lung tumors.9 MRgRT substantially improves tumor and normal tissue visualization allowing for greater delineation of radiation targets vs OARs.10 Furthermore, MRgRT permits online adaptive RT, which is the process of optimizing the initial radiation treatment plan based on “anatomy of the day” obtained from daily on-board MRI imaging. Thus, MRgRT could be utilized to adapt treatment plans to ensure that OAR constraints are met during each fraction. MRgRT also allows for continuous, real-time imaging during radiation delivery, which allows for tracking of intrafraction tumor motion due to respiration.
In addition to motion management, real-time imaging provides assurance that adequate dose was delivered to the target and that OARs were appropriately spared. Currently, 2 MRgRT devices for treating patients are commercially available, the ViewRay MRIdian and Elekta Unity.
Comparison of the technical specifications are illustrated in Table 1. Granted, as one of the first thoracic cases treated with an MRgRT approach at our institution, as well as some of the current technical limitations of the device in terms of active motion management, this case is intended to illustrate the workflow, image quality, and potential for use of this technology in the future to improve upon outcome for treatment of thoracic malignancies with MRgRT. We intend to treat other patients with central/ultracentral lung tumors with a similar workflow as part of a prospective phase I clinical trial.
Although there are multiple ongoing clinical trials of CT-based SBRT for central lung tumors, few studies have evaluated the role of MRgRT with daily adaptive plans for these patients. An initial simulation trial of MR-guided hypofractionated RT for 12 central thoracic tumors demonstrated improved PTV coverage and OAR sparing with use of midtreatment adaptive planning.11 This was followed by a phase I trial of stereotactic MRgRT using online adaptive RT for ultracentral thoracic tumors.12 In this study, 5 patients underwent MRgRT with 50 Gy/5 fractions and 4 patients required at least 1 adapted fraction to either improve PTV coverage or reverse OAR violations. At 6 months, local control was 100% and there were no grade ≥ 3 toxicities. A larger, retrospective study reviewed outcomes using stereotactic MRgRT for 50 consecutive, high-risk thoracic tumors (central location, prior RT, and interstitial lung disease).13 This study reported low rates of toxicity (grade ≥ 3 8%) and 1-year local control of 96%. Overall, the aforementioned trials demonstrate the feasibility and potential benefit of MRgRT. Notably, they used the ViewRay MRIdian, which is a low-field MR device as opposed to high-field MR with the Elekta Unity.
As the MR-linac is a relatively new device, drawbacks remain to MRgRT such as dosimetric impact of magnetic field effects on secondary electrons, suboptimal imaging of small parenchymal lesions due to low proton density of lungs, and the need for optimization of real-time motion management. To better characterize the potential benefit of MRgRT for patients with central NSCLC, we plan to conduct a 2-part clinical trial of MRgRT for patients with central NSCLC. First, we will assess the potential dosimetric benefit of MRgRT with simulated online adaptive planning. The initial cohort of patients will undergo daily onboard MR imaging that will be used to simulate adaptive plans, which will be compared to the actual delivered plan. Clinical and imaging characteristics will be assessed to identify patients most likely to benefit from MRgRT. The second cohort of patients will undergo treatment on the MR linac with online adaptive planning, reduction of fraction numbers from hypofractionation to SBRT whenever feasible, and prioritizing strict daily fractional OAR doses for each fraction. Given the advantages of MRgRT, we hypothesize that MRgRT will permit delivery of high biologically effective dose (BED) for central tumors without excessive rates of toxicity.
Conclusion
Oligometastases are common for patients with NSCLC and local consolidation therapy with radiation may be an effective treatment strategy. However, central lung cancers remain a challenging tumor site given the close proximity to multiple critical OARs. MRgRT provides several advantages over conventional CT-based treatment including improved tumor delineation, potential for daily adaptive plans, and real-time imaging. These benefits may improve the therapeutic ratio of RT and enable achievement of high BED without severe toxicity. Further investigation is warranted, and we plan to conduct a clinical trial of MRgRT for central lung cancer at our institution.
References
- Gomez DR, Tang C, Zhang J, et al. Local consolidative therapy vs. maintenance therapy or observation for patients with oligometastatic non-small-cell lung cancer: long- term results of a multi-institutional, phase II, randomized study. J Clin Oncol. 2019;37(18):1558-1565.
- Hissoiny S, Ozell B, Bouchard H, Després P. GPUMCD: a new GPU-oriented Monte Carlo dose calculation platform. Med Phys. 2011;38(2):754-764.
- Ger RB, Yang J, Ding Y, et al. Accuracy of deformable image registration on magnetic resonance images in digital and physical phantoms. Med Phys. 2017;44(10):5153-5161.
- Yang J, Vedam S, Lee B, et al. Online adaptive planning for prostate stereotactic body radiotherapy using a 1.5 Tesla magnetic resonance imaging-guided linear accelerator. Phys Imaging Radiat Oncol. 2021;17:20-24.
- Lievens Y, Guckenberger M, Gomez D, et al. Defining oligometastatic disease from a radiation oncology perspective: an ESTRO-ASTRO consensus document. Radiother Oncol. 2020;148:157-166.
- Bergsma DP, Salama JK, Singh DP, Chmura SJ, Milano MT. Radiotherapy for oligometastatic lung cancer. Front Oncol. 2017;7:210.
- Palma DA, Olson R, Harrow S, et al. Stereotactic ablative radiotherapy for the comprehensive treatment of oligometastatic cancers: long-term results of the SABR-COMET phase II randomized trial. J Clin Oncol. 2020;38(25):2830-2838.
- Henke L, Kashani R, Yang D, et al. Simulated online adaptive magnetic resonance-guided stereotactic body radiation therapy for the treatment of oligometastatic disease of the abdomen and central thorax: characterization of potential advantages. Int J Radiat Oncol Biol Phys. 2016;96(5):1078-1086.
- Padgett KR, Simpson GN, Llorente R, Samuels MA, Dogan N. Feasibility of adaptive MR-guided stereotactic body radiotherapy (SBRT) of lung tumors. Cureus.2018;10(4):e2423.
- Noel CE, Parikh PJ, Spencer CR, et al. Comparison of onboard low-field magnetic resonance imaging versus onboard computed tomography for anatomy visualization in radiotherapy. Acta Oncol. 2015;54(9):1474-1482.
- Henke LE, Kashani R, Hilliard J, et al. In silico trial of MR-guided midtreatment adaptive planning for hypofractionated stereotactic radiation therapy in centrally located thoracic tumors. Int J Radiat Oncol Biol Phys. 2018;102(4):987-995.
- Henke LE, Olsen JR, Contreras JA, et al. Stereotactic MR-guided online adaptive radiation therapy (SMART) for ultracentral thorax malignancies: results of a phase 1 trial. Adv Radiat Oncol. 2019;4(1):201-209.
- Finazzi T, Haasbeek CJA, Spoelstra FOB, et al. Clinical outcomes of stereotactic MR-guided adaptive radiation therapy for high-risk lung tumors. Int J Radiat Oncol Biol Phys. 2020;107(2):270-278.
Citation
Weng J, Odwuor A, Yang J, Lee P. High-Field MR-Guided Radiation Therapy for Oligometastatic Central Lung Cancer: Current State and Future Opportunities. Appl Rad Oncol. 2021;(4):40-47.
December 28, 2021