Impact of irradiation protocol deviations on the outcome of unresectable stage III NSCLC patients receiving concurrent chemoradi
Images
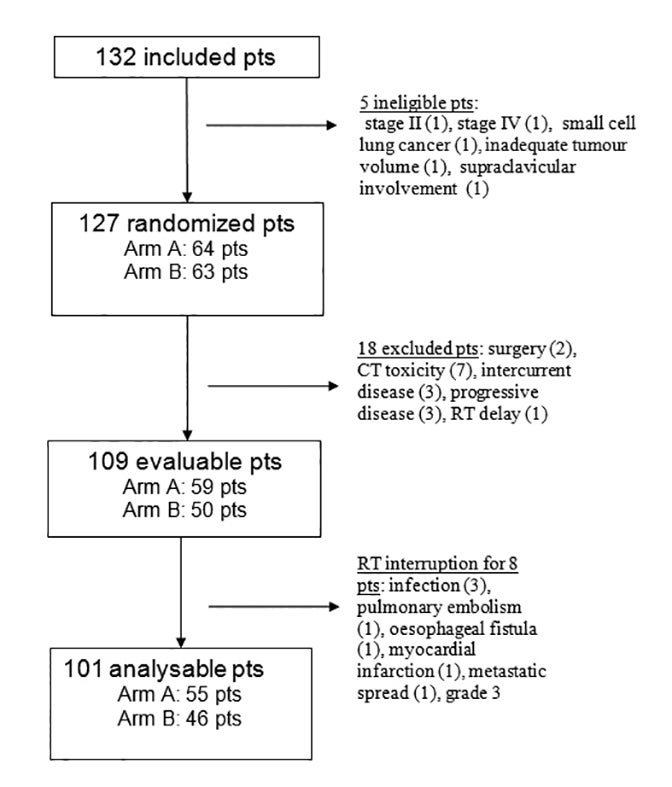
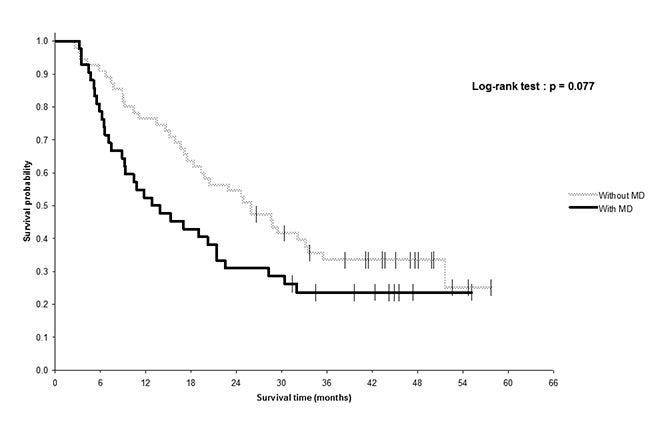
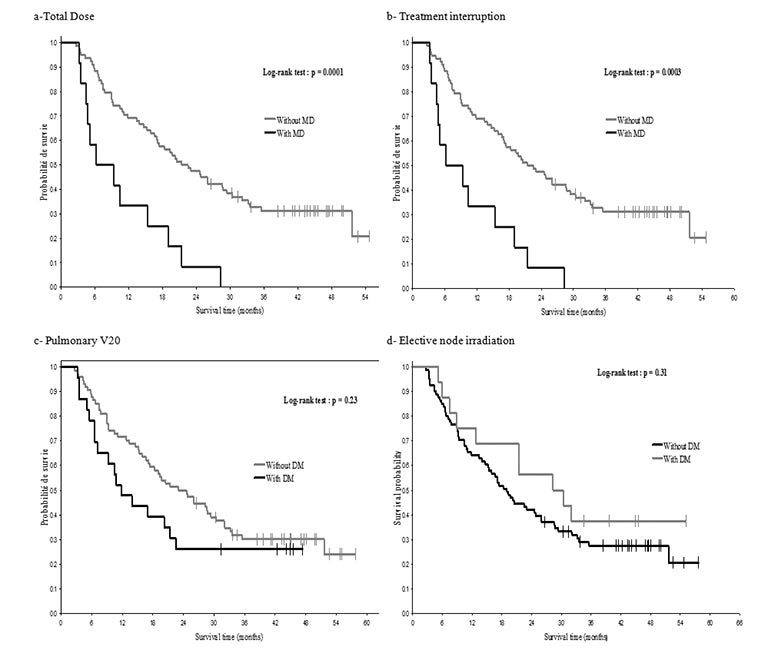
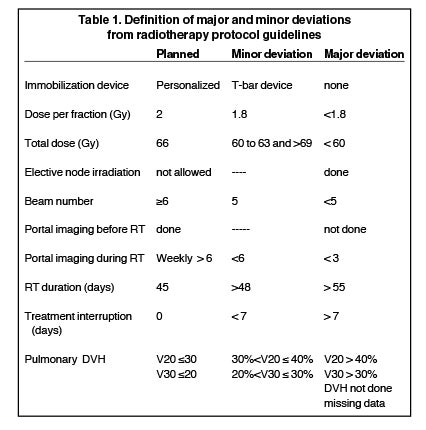
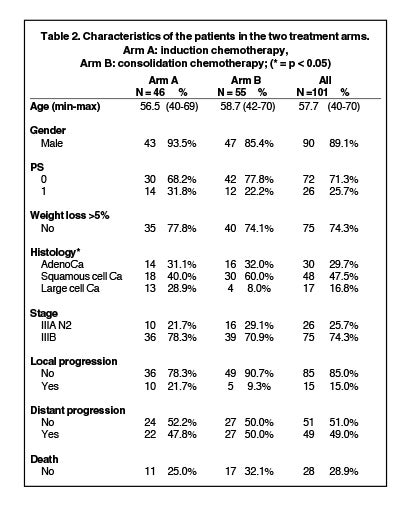
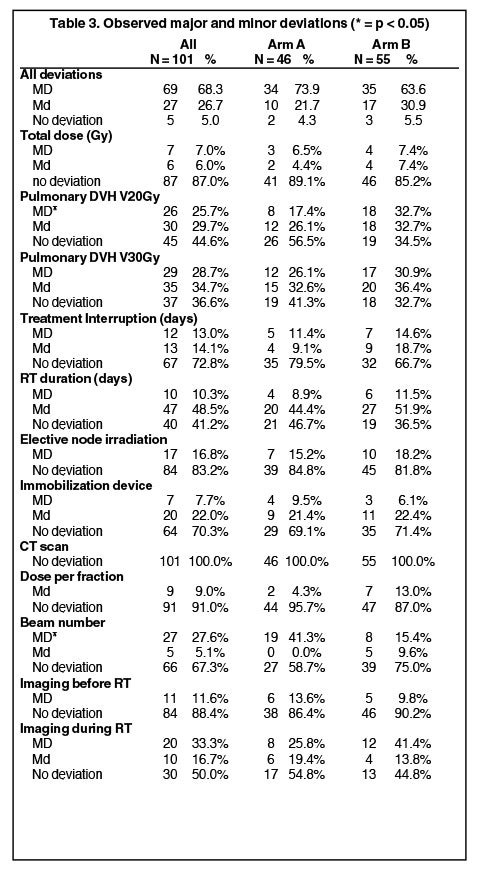
A dose-effect relationship of exclusive radiotherapy (RT) is likely to exist in non-small cell lung cancer (NSCLC) with lower doses providing poorer local control.1,2 However, high doses result in more severe toxicity. Based on 3D conformal RT, thresholds have been established for pulmonary dose-volume histograms (DVH), with the aim of avoiding severe radio-induced pneumonitis.3 In selected patients with unresectable stage III NSCLC, concomitant chemoradiation has proven superior to sequential combination therapy,4,5 but causes more frequent acute esophagitis.6-9 Therefore, RT quality control is useful for reducing treatment toxicity and improving tumor control.
In some other malignancies, both toxicity and overall survival (OS) correlate with the quality of RT.10 Very few data on RT quality are available for NSCLC, as quality assurance usually focuses on technical and physical assessment of linear accelerators.11-12
We analyzed RT quality in a phase II randomized trial of concurrent chemoradiation for unresectable stage III NSCLC. The main objectives were to assess compliance with the trial’s protocol and the impact of observed deviations on survival and toxicity.
Materials and methods
The GLOT-IFCT-GFPC 02.01 (Groupe Lyon-Saint-Etienne d’Oncologie Thoracique, Intergroupe Francophone de Cancérologie Thoracique, Groupe Français de Pneumo-Cancérologie), study was a multicenter randomized phase II trial of concurrent chemoradiation either preceded (arm A) or followed (arm B) by chemotherapy for unresectable stage III NSCLC. The chemotherapy regimen was cisplatin 80 mg/m² and paclitaxel 200 mg/m² every 21 days for two cycles, cisplatin 80 mg/m² every 21 days, and vinorelbine 15 mg/m² weekly concurrently. Standard inclusion criteria applied.
The trial’s RT protocol conformed to European Organisation for Research and Treatment of Cancer (EORTC) guidelines.13 The gross tumor volume (GTV) included the primary tumor and enlarged mediastinal lymph nodes (smallest diameter ≥ 1 cm). Prophylactic node irradiation was not authorized. The planning treatment volume (PTV) was defined as GTV + 15 mm without field reduction. A personalized immobilization device was required for CT scanning in the treatment position. The total dose was 66 Gy in 33 fractions. Three-dimensional conformal irradiation was mandatory. Maximum spinal cord dose was 46 Gy. Pulmonary DVH were required, with the following recommended values: V20 ≤ 30% and V30 ≤ 20%. Six beams or more had to be used. All fields were verified before starting treatment, and weekly thereafter. All patients were examined weekly by the radiation oncologist, who scored acute toxicity according to the CTCAE v3 scale. The following items were collected and reviewed twice a year by the GFPC radiation oncologists panel: immobilization device, total dose, dose per fraction, prophylactic nodal irradiation (supraclavicular or mediastinal), number of beams, control imaging before and during irradiation, treatment duration, treatment interruption (number of days, reasons), and pulmonary DVH.
As the aim of this study was to assess the quality of RT, the analysis included only patients who completed the concurrent chemoradiation. Major (MD) and minor (md) deviations were defined for 11 criteria (Table 1). Four clinically most relevant MDs were grouped together for analysis: the total dose, the pulmonary V20 DVH (V20), treatment interruption and elective node irradiation.
Statistical analysis
Comparisons were made using the chi-2 or Wilcoxon test. The survival time was calculated from the date of cancer diagnosis to the date of death, or censored at the date of last follow-up for survivors, based on Kaplan-Meier estimates.14 Progression-free survival was calculated from the date of cancer diagnosis to the date of progression. Cox proportional hazards models were used to test the effect of each deviation, with adjustment for the treatment arm.
Results
Between May 2002 and March 2005, 132 patients were irradiated in 28 centers. Median follow up was 44.9 months (95% CI: 42.3-47.4). Five patients were ineligible, 18 patients were excluded before concomitant treatment, and 8 patients did not receive the entire irradiation. The remaining 101 patients completed the concurrent chemoradiation and constituted the study population (Figure 1). The characteristics of the patients were well-balanced between the two arms, except for the histological type (Table 2).
Grade 1-2 pulmonary toxicity affected 24% and 25% of the patients, respectively, in arm A and arm B; one case of grade 5 pulmonary toxicity occurred in arm B. Grade 3-4 esophageal toxicity affected 15.8% of patients overall (6 patients in arm A, 10 patients in arm B). The 2-year OS rates were 47% and 43% in arms A and B, respectively, and the objective response rates were 55% and 48%.
Full data on RT were provided by all but 3 of the radiotherapy centers (6 patients). All patients received an adequate photon energy, and most patients received 2-Gy fractions. Twelve patients (13%) had a treatment interruption of one week or more.
Among the 101 assessable patients, 69 (68.3%) had at least one MD, 27 (26.7%) had at least one md, and 5 (5%) had no deviation (Table 3).
The most frequent MDs were an inappropriate number of beams in 27 (27.6%) cases, and inadequate values for pulmonary DVH, respectively, in 26 patients (25.7%) for V20, and 29 patients (28.7%) for V30. Other MDs were prophylactic nodal irradiation and treatment interruption lasting >1 week. The total dose was that recommended, except in 7 patients. There was a strong correlation between the total dose and treatment interruption (p < 0.0001). Age, gender, weight loss, histological type and tumor stage were not predictive of MD risk.
There was a significant difference between the 2 treatment arms with respect to V20 MDs (17.4% in the induction arm versus 32.7% in the consolidation arm, p = 0.04). The reduction in tumor volume after 2 cycles of induction chemotherapy may explain this difference. There was no difference between the treatment arms with respect to the total dose, RT duration or elective nodal irradiation.
Consequences of the deviations
The 2-year OS tended to be lower in patients with at least one MD (40%, 95% CI: 28.1; 51.9) than in patients with no MD (53.1%, 95% CI: 35.8; 70.4), as well as the median OS (19 versus 25.3 months, p = 0.31), but the difference was not statistically significant. When considering only the 4 clinically relevant MDs (total dose, V20, treatment interruption, elective nodal irradiation), outcome tended to be poorer among patients with MDs than in patients without MD: The median OS times were respectively 13.3 and 19 months, and the 2-year OS rates were respectively 31% (95% CI: 17.8; 45) and 54.5% (95% CI: 10.6; 66.6) (p = 0.077, Figure 2). OS was significantly affected by deviations from the total dose (p = 0.0001) and by treatment interruption (p = 0.0003), but not by V20 or elective nodal irradiation (Figure 3). Patients with MD from the total dose had a median OS of 4.8 months, compared to 23 months in other patients, and the difference in the 2-year OS rate was huge (0% versus 47.8%, 95% CI: 37.2; 57.6). Patients with MDs due to treatment interruption received a lower total dose (54.7 Gy vs 66 Gy), which likely influenced their OS. In multivariate analysis, the only factor predictive for a lower OS was TI.
There was no difference in progression-free survival or the time to local progression according to the MDs. Metastasis-free survival was significantly shorter in patients with MDs for treatment duration (p = 0.004).
Discussion
The study’s aim was to assess the quality of RT and its impact on patient outcome. In published trials of concurrent chemoradiation, information on the RT is restricted to the total dose, fractionation and recommended volumes.8 Data on the treatment actually administered are rarely provided.6,7,9 In definitive radiotherapy, the volumes, total dose and toxicity are strongly related. As radiotherapy plays a major role in the local control of unresectable NSCLC, the question arises as to whether the quality of radiotherapy is related to patient outcome. Despite protocol requirements concerning centralized review of the radiotherapy data, some centers failed to provide their patients’ records, or provided only very sketchy information with many missing data.
The OS time in the entire study population was slightly longer than in other series of concurrent chemoradiation for NSCLC, with a median of 20.2 months, compared to 15 months in the French GFPC 95.01 study,7 17.1 months in the Radiation Therapy Oncology Group (RTOG) 94.10 study,15 and 16.5 months in the Japanese trial16 and the EORTC study.6 OS was significantly influenced by the total dose (4.8 months if < 60 Gy vs 21.9 months if ≥ 60 Gy, p < 0.0001), as in previous studies that showed that the total dose must be > 60 Gy for curative purposes.1,2
OS was also negatively affected by treatment interruption and by a longer radiotherapy duration (p = 0.005 and p = 0.0001, respectively), as was metastasis-free survival (p = 0.047 and p = 0.002, respectively). Treatment interruption is a well-known prognostic factor in patients with head-and-neck tumors or cervical cancer.17,18 Fowler suggested that clonogens proliferated within the tumor after 3 or 4 weeks of radiotherapy.19 Machtay et al.20 pooled patient data from 3 RTOG trials, including concurrent chemoradiation for unresectable NSCLC, with most patients receiving hyperfractionated radiotherapy. Altogether, 18% of the patients had treatment interruption lasting more than 5 days. The median OS was not significantly better among patients who completed their treatment on time (19.5 versus 14.8 months, p = 0.15).
In multivariate analysis, prolonged treatment time was associated with poorer OS (hazard ratio 1.02, CI: 1.003-1.03, p = 0.01) and a lower total dose (p = 0.03). In the present study, there was also a strong correlation between treatment interruption and total dose. In the 12 patients who had MD for treatment interruption, the mean total dose was 54.7 Gy, compared to 66 Gy among patients with no delay (p < 0.0001). Progression-free survival and locoregional progression-free survival were not affected by the occurrence of MDs, suggesting that the impaired survival reported in the deviation group was not related to an increased rate of locoregional recurrence. Treatment interruption (p = 0.047) and longer RT duration (p = 0.002) were associated with a poorer metastasis-free survival. The small size of this study probably explains the lack of any significant difference in OS according to the occurrence of any MD or any of the 4 clinically relevant MDs.
Prophylactic nodal irradiation was delivered to 17 (16.8%) patients, either to uninvolved mediastinal areas or to the supraclavicular fossae. Some studies have shown no improvement in local control or survival with elective node irradiation.21,22 In the present study, patients receiving prophylactic node irradiation did not have worse pulmonary DVH values, and were not at a greater risk of receiving an inadequate total radiation dose. This could be explained by the use of field reduction techniques to avoid excessive irradiated volumes, and by the central location of the node areas in the chest. Pulmonary DVH values for V20 and V30 were extremely variable, ranging from 9% to 64% and from 3% to 61%, respectively. For V20, the median value was 31.4% and the mean value was 30.5%. According to the protocol, the percentage of lung receiving more than 20 Gy should not have exceeded 30%, which is a stricter cut-off than the 35% accepted in most recent studies. Nevertheless, MD from V20 was defined as V20 > 40%, which is well above the maximal value recommended. After excluding missing values (11 patients), 24 (23.8%) patients had V20 > 35% and 15 (16.7%) patients had V20 > 40%. The rates of MD for the total dose (7%) and pulmonary DVH (25.7%) seem to show that radiation oncologists preferred to stick to the recommended dose while accepting inadequate pulmonary DVH values.
The question of whether the PTV, the GTV, or neither should be subtracted from the total lung volume when calculating DVH values is controversial, and practices vary across published studies.13 The protocol guidelines required the PTV to be subtracted from the total volume of the two lungs. Despite the large number of patients with DVH values outside the recommended range, this deviation did not correlate with increased toxicity. The incidence of acute pulmonary toxicity was low and similar to that reported in randomized trials of concurrent chemoradiation and in the Cochrane review5,7,15,16 A Japanese study focusing on radio-induced pneumonitis following concurrent chemoradiation showed a 28% crude incidence of grade 2 or higher pneumonitis. Severe toxicity (grade ≥ 3) affected 4.2% of patients.23 This confirms that radiation pneumonitis may be overlooked unless specifically sought.
In a recent retrospective study focusing on dosimetric factors associated with treatment-related pneumonitis in patients with NSCLC receiving concurrent chemoradiation, the cumulative rate of severe pneumonitis was 22% at 6 months and 32% at 12 months, which is higher than the rates usually reported. The authors found that V5 (the percentage of lung volume receiving 5 Gy) was the most relevant factor for predicting pulmonary toxicity, with pneumonitis incidence rates of 3% and 38% for V5 ≤ 42% and > 42%, respectively. The influence of the mean lung dose was confirmed, with a threshold of 16.5 Gy. Interestingly, the patients analyzed had mainly received platinum-taxane or platinum-etoposide combinations and, occasionally, irinotecan, gemcitabin or doxorubicin-based regimens, which are known to be highly radiosensitizing and could explain the high rates of severe pulmonary toxicity.24 Concurrent chemoradiation with cisplatin and vinorelbine is usually better tolerated than other chemotherapy regimens,8 as confirmed in this trial. In a literature-based review of clinically relevant radiation pneumonitis following concurrent chemoradiation for lung carcinoma, the rate of severe radiation pneumonitis was 7.8% and the only factor associated with an increased incidence of toxicity was a fraction size larger than 2.67 Gy.25
In a more recent retrospective study, the incidence of severe radiation pneumonitis was similar (8.3%), and the only factors associated with a higher incidence were performance status (1 versus 0) and female gender.26 The number of events suggestive of severe radiation pneumonitis was insufficient to test these hypotheses. Unfortunately, the protocol was not designed to collect treatment planning volumes, and it was not therefore possible to study correlations between these volumes and toxicity, survival or loco-regional control.
Conclusion
This study shows an association between poorer overall survival and prolonged irradiation in a homogenous group of patients treated with concurrent chemoradiation and conventional fractionation. This calls for procedures to obtain treatment planning data before randomization, in order to include only patients whose V20 pulmonary DVH is no more than 35%. The next step will be to organize a centralized review of radiotherapy quality criteria and protocol compliance before participating radiation oncologists are allowed to enroll patients, as is already the case in some multicenter studies.
References
- Perez CA, Pajak TF, Rubin P, et al. Long-term observations of the patterns of failure in patients with unresectable non-oat cell carcinoma of the lung treated with definitive radiotherapy. Report by the Radiation Therapy Oncology Group. Cancer. 1987;59:1874-1881.
- Rengan R, Rosenzweig KE, Venkatraman E, et al. Improved local control with higher doses of radiation in large-volume stage III non-small-cell lung cancer. Int J Radiat Onco Biol Phys. 2004;60:741-747.
- Graham MV, Purdy JA, Emami B, et al. Clinical dose-volume histogram analysis for pneumonitis after 3D treatment for non-small cell lung cancer (NSCLC). Int J Radiat Oncol Biol Phys. 1999;45:323-329.
- Auperin A, Rolland E, Curran W. Concomitant radio-chemotherapy (RT-CT) versus sequential RT-CT in locally advanced non-small cell lung cancer (NSCLC): A meta-analysis using individual patient data from randomized trials. J Thorac Oncol. 2007;2:AbstractA1-05,S 310.
- Rowell NP, O’rourke NP. Concurrent chemoradiotherapy in non-small cell lung cancer. Cochrane Database Syst Rev. 2004;CD002140.
- Belderbos J, Uitterhoeve L, van Zandwijk N, et al. Randomised trial of sequential versus concurrent chemo-radiotherapy in patients with inoperable non-small cell lung cancer (EORTC 08972-22973). Eur J Cancer 2007;43:114-121.
- Fournel P, Robinet G, Thomas P, et al. Randomized phase III trial of sequential chemoradiotherapy compared with concurrent chemoradiotherapy in locally advanced non-small-cell lung cancer: Groupe Lyon-Saint-Etienne d’Oncologie Thoracique-Groupe Francais de Pneumo-Cancerologie NPC 95-01 Study. J Clin Oncol. 2005;23:5910-5917.
- Vokes EE, Herndon JE, Crawford J, et al. Randomized phase II study of cisplatin with gemcitabine or paclitaxel or vinorelbine as induction chemotherapy followed by concomitant chemoradiotherapy for stage IIIB non-small-cell lung cancer: cancer and leukemia group B study 9431. J Clin Oncol. 2002;20:4191-4198.
- Zatloukal P, Petruzelka L, Zemanova M, et al. Concurrent versus sequential chemoradiotherapy with cisplatin and vinorelbine in locally advanced non-small cell lung cancer: a randomized study. Lung Cancer. 2004;46:87-98.
- Carrie C, Hoffstetter S, Gomez F, et al. Impact of targeting deviations on outcome in medulloblastoma: study of the French Society of Pediatric Oncology (SFOP). Int J Radiat Oncol Biol Phys. 1999;45:435-439.
- Aird EG, Williams C, Mott GT, et al. Quality assurance in the CHART clinical trial. Radiother Oncol. 1995;36:235-244.
- Kouloulias VE, Poortmans PM, Bernier J, et al. The Quality Assurance programme of the Radiotherapy Group of the European Organization for Research and Treatment of Cancer (EORTC): a critical appraisal of 20 years of continuous efforts. Eur J Cancer. 2003;39:430-437.
- Senan S, De Ruysscher D, Giraud P, et al. Literature-based recommendations for treatment planning and execution in high-dose radiotherapy for lung cancer. Radiother Oncol. 2004;71:139-146.
- Kaplan EL and Meier P. Nonparametric estimation from incomplete observations. J Am Stat Assoc. 1958;53:457-481.
- Curran WJ, Scott CB, Langer CJ. Long term benefit is observed in a phase III comparison of sequential vs concurrent chemo-radiation for patients with unresected satge III nsclc: RTOG 9410. Proc Am Soc Clin Oncol. 2003;22:621 (abstract 2499).
- Furuse K, Fukuoka M, Kawahara M, et al. Phase III study of concurrent versus sequential thoracic radiotherapy in combination with mitomycin, vindesine, and cisplatin in unresectable stage III non-small-cell lung cancer. J Clin Oncol. 1999;17:2692-2699.
- Perez CA, Grigsby PW, Castro-Vita H, et al. Carcinoma of the uterine cervix. I. Impact of prolongation of overall treatment time and timing of brachytherapy on outcome ofradiation therapy. Int J Radiat Oncol Biol Phys 1995;32:1275-1288.
- Robertson AG, Robertson C, Perone C, et al. Effect of gap length and position on results of treatment of cancer of the larynx in Scotland by radiotherapy: a linear quadratic analysis. Radiother Oncol 1998;48:165-173.
- Fowler JF, Chappell R. Non-small cell lung tumors repopulate rapidly during radiation therapy. Int J Radiat Oncol Biol Phy.s 2000;46:516-517.
- Machtay M, Hsu C, Komaki R, et al. Effect of overall treatment time on outcomes after concurrent chemoradiotherapy for locally advanced non-small-cell lung carcinoma: analysis of the Radiation Therapy Oncology Group (RTOG) experience. Int J Radiat Oncol Biol Phys. 2005;63:667-671.
- Senan S, Burgers S, Samson MJ, et al. Can elective node irradiation be omitted in stage III non-small-cell lung cancer? Analysis of recurrences in a phase II study of induction chemotherapy and involved-field radiotherapy. Int J Radiat Oncol Biol Phys. 2002;54:999-1006.
- Rosenzweig KE, Sura S, Jackson A, et al. Involved-field radiation therapy for inoperable non small-cell lung cancer. J Clin Oncol. 2007;25:5557-5561.
- Tsujino K, Hirota S, Endo M, et al. Predictive value of dose-volume histogram parameters for predicting radiation pneumonitis after concurrent chemoradiation for lung cancer. Int J Radiat Oncol Biol Phys. 2003;55:110-115.
- Wang S, Liao Z, Wei X, et al. Analysis of clinical and dosimetric factors associated with treatment-related pneumonitis (TRP) in patients with non-small-cell lung cancer (NSCLC) treated with concurrent chemotherapy and three-dimensional conformal radiotherapy (3D-CRT). Int J Radiat Oncol Biol Phys. 2006;66:1399-1407.
- Roach M, III, Gandara DR, Yuo HS, et al. Radiation pneumonitis following combined modality therapy for lung cancer: analysis of prognostic factors. J Clin Onco.l 1995;13:2606-2612.
- Robnett TJ, Machtay M, Vines EF, et al. Factors predicting severe radiation pneumonitis in patients receiving definitive chemoradiotherapy for lung cancer. Int J Radiat Oncol Biol Phys. 2000;48:89-94.