Integrating MR-Guided Radiation Therapy Into Clinical Practice: Clinical Advantages and Practical Limitations
Images
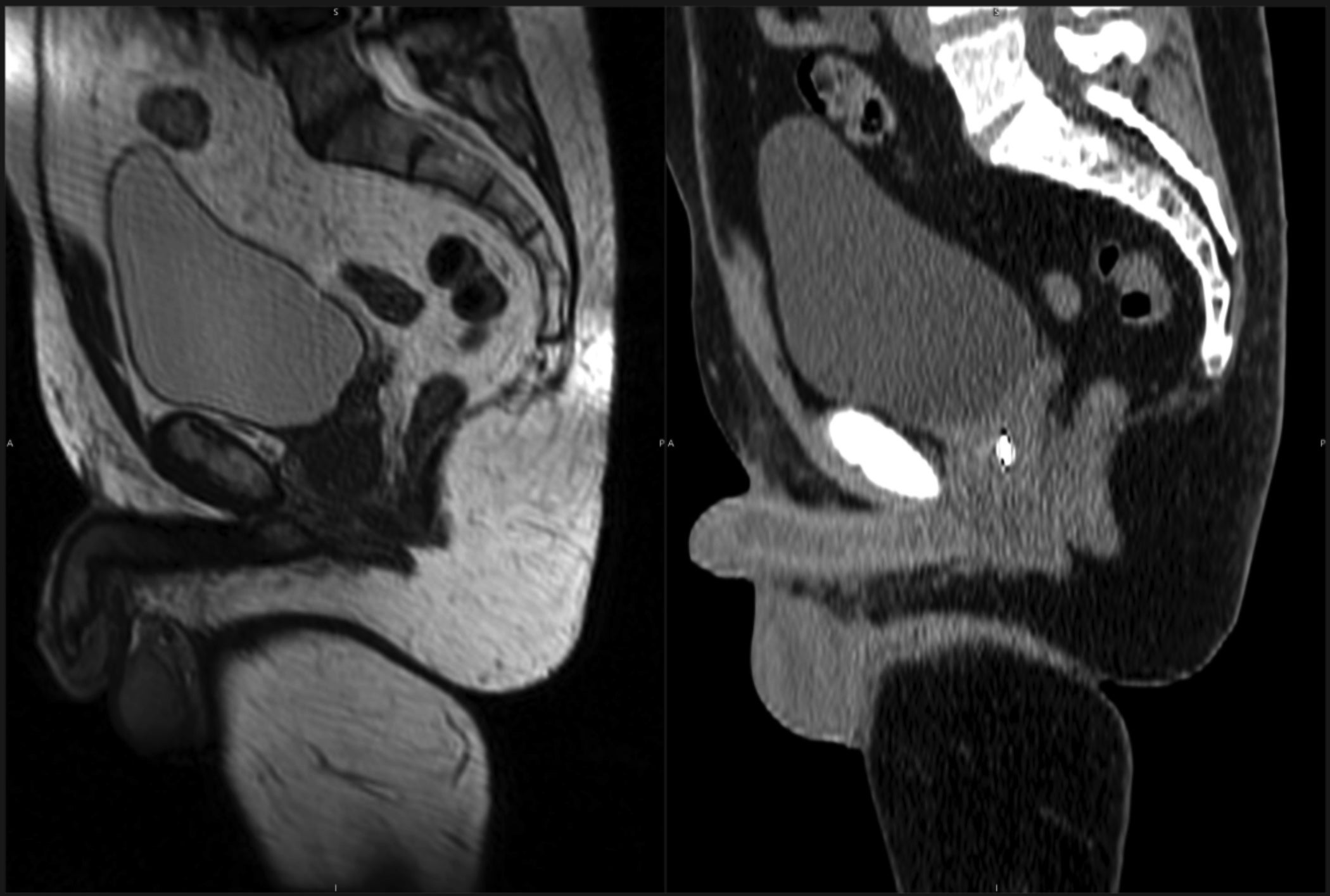
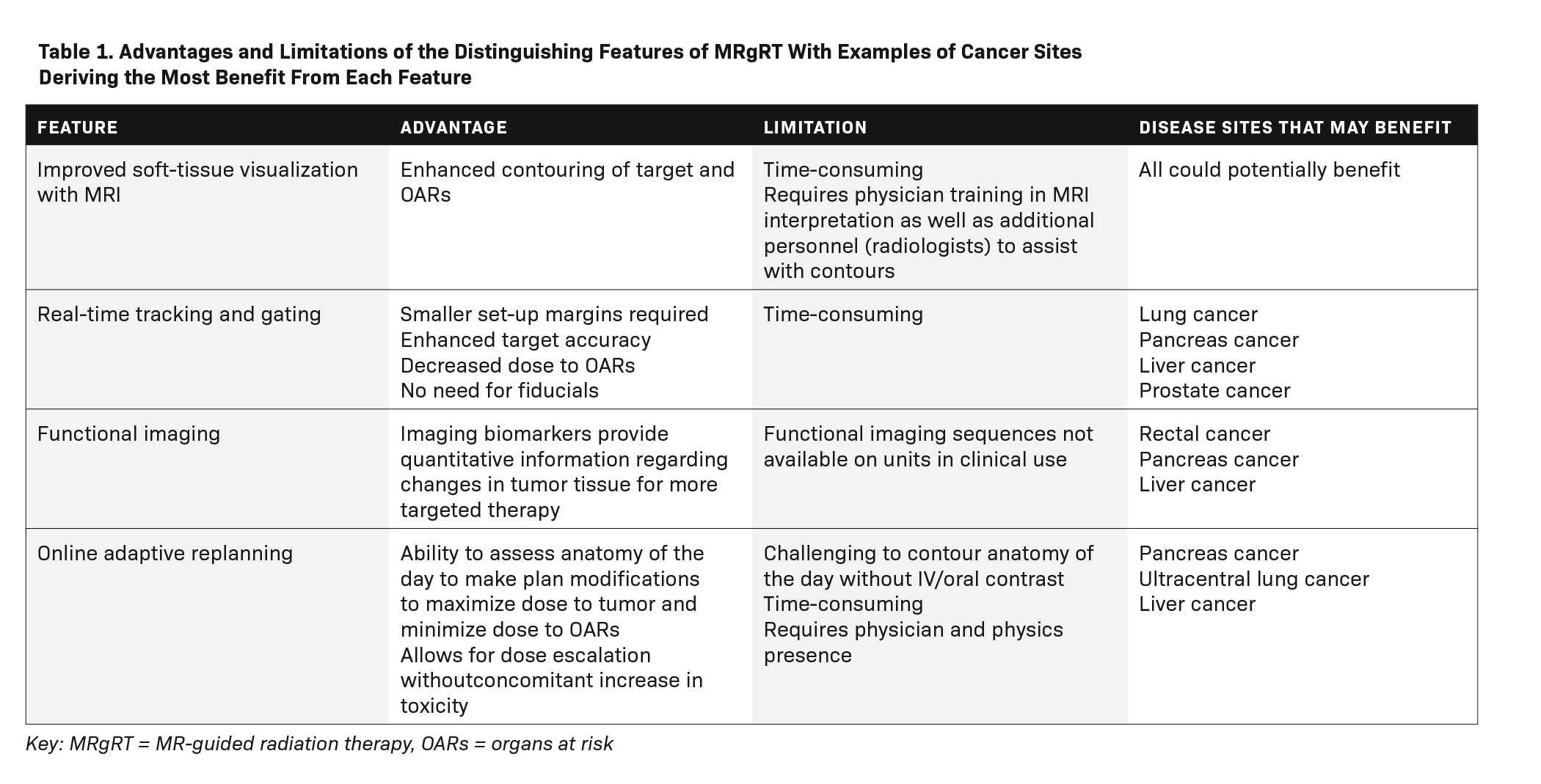
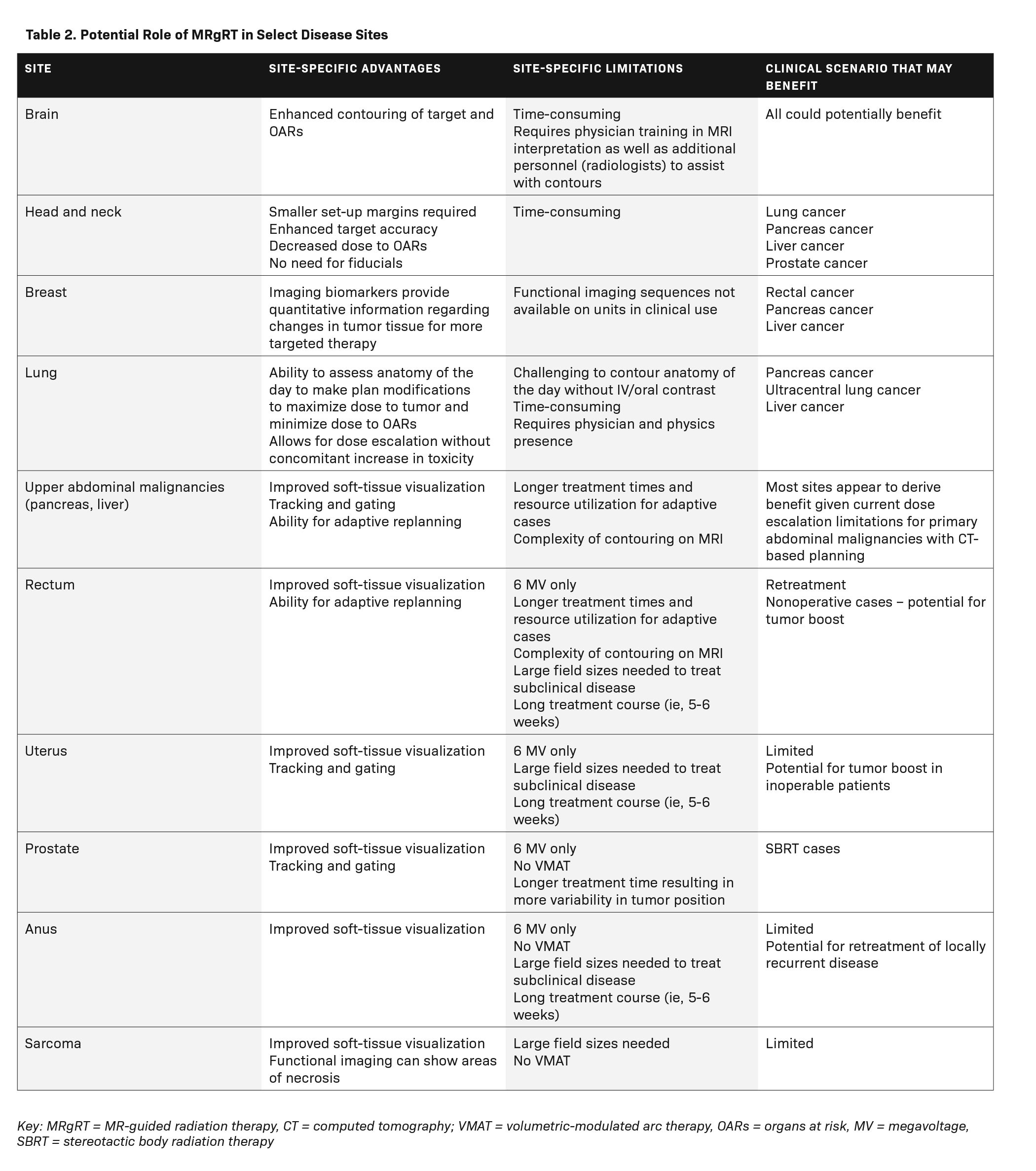
SA-CME credits are available for this article here.
MR-guided radiation therapy (MRgRT) has emerged as a promising radiation treatment modality for a variety of solid malignancies. This technology is being increasingly adopted at practices across the US and abroad. ViewRay, the company to introduce the first MRgRT system into clinical practice at Washington University in 2014,1 now has 45 treatment units installed at practices around the world. Meanwhile, Elekta developed the Unity system which first came into clinical practice at UMC Utrecht in the Netherlands in 2017,2 and is now installed in 25 practices worldwide. While there are a number of benefits to MRgRT, it is a costly and resource-intensive investment that can strain radiation oncology clinics not adequately prepared to incorporate this new treatment modality into practice. The purpose of the present work is to review both the clinical advantages and the practical limitations of MRgRT, and to suggest which patients are likely to derive the greatest benefit from this technology. We aim to provide a practical guide for centers acquiring MRI-based linear accelerators (MR-linacs) to incorporate the technology more seamlessly into clinical practice.
Clinical Advantages of MRgRT
There are several advantages to MRgRT over conventional computed tomography (CT)-guided treatment techniques. Among these advantages are superior target visualization through improved soft-tissue imaging, real-time tumor tracking, real-time image-guided gating, and real-time plan adaptation (Table 1).3-5 Improved soft-tissue imaging has been cited as a primary reason patients are treated on an MR-linac rather than a CT-based machine.6 A comparison of pelvic anatomy acquired on a CT-simulator vs an MR-simulator is shown in Figure 1.
Daily MRI acquisition on an MR-linac allows for superior organ-at-risk and target-volume visualization, which, in turn, allows for plan adaptation.5 Online adaptive radiation therapy can improve planning target volume (PTV) coverage and reduce treatment toxicity.7-9 In their study of 10 patients undergoing pancreas stereotactic body radiation therapy (SBRT), El-Bared et al showed that adaptive replanning resulted in a 10% improvement in the volume of the PTV receiving 100% of the prescribed dose (90% vs 80%, P < 0.01) compared with nonadaptive plans.7 The maximum dose to the duodenum was achieved more frequently in the adaptive vs nonadaptive plans.7
By allowing for improved target volume coverage with reduced toxicity, online adaptive replanning permits dose escalation. In their trial of MR-guided online adaptive radiation therapy (SMART) for abdominal malignancies, Henke et al found that plan adaptation resulted in improved PTV coverage in 64 of 97 delivered fractions, with zero Grade 3 or higher toxicity.10 Ablative doses of radiation could only be delivered with adaptive replanning, permitting the delivery of higher radiation doses than has historically been possible to tumors within the abdomen.11 The ability to dose escalate using adaptive replanning on an MR-linac for inoperable pancreatic cancer has also been demonstrated.12 Henke et al also conducted a phase I trial of SMART for ultracentral thoracic malignancies and found that PTV coverage was improved in 30% of fractions with adaptive replanning.13 The authors found they were able to reverse potentially life-threatening severe toxicities observed in historical trials of SBRT for ultracentral thoracic malignancies, which has precluded the use of ablative SBRT for many of these cases.
While online adaptive radiation therapy is an attractive feature of the MR-linac, it is not utilized for every patient treated on these machines. Reporting on their first 2.5 years of treating patients on the world’s first MR-linac, Fischer-Valuk et al note that only about 25% of patients were treated with online adaptive radiation therapy.6 The majority of these patients had abdominal malignancies. Among the patients treated with 5-fraction SBRT adaptive radiation, the incidence of plan adaption was 84%, which is in keeping with other reports in the literature.14
Additional advantages of the MR-linac include real-time tumor tracking and gating. Unlike gating on a conventional linac, gating on an MR-linac does not require fiducials or increase radiation exposure to the patient. The two-dimensional cine imaging on an MR-linac provides real-time live cine MRI frames that can be deformably registered to a preview cine MRI scan acquired right before the start of treatment.15 A boundary is created to identify the tracking margin and radiation is halted whenever the anatomy of interest moves outside the boundary. This allows for a reduction in the margin typically added for setup uncertainty and intrafraction motion, allowing for improved target accuracy and decreased doses to adjacent organs at risk.13,16-18
MRI-based radiation treatment delivery also can provide functional imaging to guide radiation treatment. Diagnostic diffusion-weighted MRI, for example, has been shown to predict response to radiation therapy for a number of disease sites.19-23 Diffusion-weighted MRI images can be used to create apparent diffusion coefficients (ADC), which provide quantitative information regarding changes in tumor tissue, such as development of necrosis, to guide therapy.24 Dynamic contrast-enhanced (DCE) MRI sequences measure tissue perfusion and vascularity, and can serve as another biomarker for radiation delivery.25 These imaging biomarkers can assist in patient selection for dose escalation or de-escalation, allowing for more individualized patient treatment.26,27 While the feasibility of obtaining functional imaging on MR-linac units has been demonstrated, it is not available for commercial use at this time.26,28,29 The modifications necessary to adapt MRI scanners for use in radiation treatment systems, such as a low field strength in the case of ViewRay’s MRIdian or the split gradient coil system in the case of Elekta’s Unity, may degrade the quality of the quantitative MRI data provided.30 Efforts are ongoing to overcome these challenges such that functional imaging can one day be widely available on MR-linac systems in use.
The many advantages of MRgRT make this an attractive technology to adopt in radiation therapy practices. The cancers seen most in radiation oncology clinics – cancers of the breast, lung, colorectum, and prostate – all appear to derive benefit from treatment on an MR-linac (Table 2). In the case of breast cancer, MRgRT has been proposed as a valuable treatment technique for patients receiving partial-breast irradiation, given that the postoperative tumor bed is more clearly visualized on MRI than on CT.31,32 Further, chest wall movement requires additional margins to account for setup uncertainty, which can be avoided with MRgRT’s real-time tumor tracking and gating.31 MRgRT has also been proposed as a solution to overcoming the challenges encountered when treating lung cancer patients by allowing for dose escalation, functional imaging, and reduced toxicity, particularly in the case of centrally located tumors.13,31,33 In the case of rectal cancer, MRgRT is an enticing solution for patients wishing to undergo organ preservation, owing to its improved soft-tissue visualization, ability for adaptive replanning, functional imaging capabilities, and tumor gating, allowing for dose escalation.27,34-36 Prostate cancer patients also appear to derive benefit from the implementation of MRgRT, given the high doses of radiation needed to treat prostate cancer while sparing the adjacent rectum and bladder, which are subject to significant variability in daily positioning.31,37,38
While there are potential advantages to treating these common malignancies with MRgRT over more conventional treatment techniques, the rarer cancers appear to derive the most benefit from this treatment (Table 2). Abdominal malignancies are challenging to treat on CT-based radiation therapy delivery systems. These cancers, including those of the pancreas and liver, are proximal to the bowel, which is intolerant of the ablative doses needed for treatment with curative intent. Moreover, abdominal organs are subject to constant fluctuations in position due to respiratory motion, gastric filling, and bowel distention. As a result, larger margins are needed to ensure adequate dose delivery to the tumor targets. A number of not only retrospective, but also prospective studies, demonstrate the safety and potential efficacy of MRgRT in managing abdominal malignancies.10,12,39-48 Recently, Hassanzadeh et al published their experience treating 44 patients with inoperable pancreatic cancer to 50 Gy in 5 fractions on an MR-linac. Local control and overall survival rates at 1 year were 84% and 68%, respectively, with low rates of late toxicity.12
Practical Limitations of MRgRT
While there are a number of advantages to MRgRT, clinicians should also consider several limitations when seeking to adopt this technology in daily practice (Table 1). Some inherent limitations associated with coupling an MRI to radiation treatment are geometric distortion, electron density disruption, susceptibility artifacts, and the inability to treat patients with contraindications to MRI, such as metal implants.18 In addition, the features that distinguish MR-based from CT-based radiation treatment – including the acquisition of MR-imaging, adaptive replanning, and gating – result in significantly longer on-table times for patients.2,10,49 MR-linacs also deliver fewer monitor units per minute and are only capable of delivering static step-and-shoot radiation plans, rather than volumetric-modulated arc therapy (VMAT), increasing beam-on time, thus further lengthening each treatment.50
There are several disadvantages to longer treatment times for cancer patients. For patients with claustrophobia, being in an enclosed space for prolonged periods can be intolerable.6,31 Patients needing to maintain a full bladder to minimize dose to organs at risk, such as rectal and prostate cancer patients, may struggle if treatment times are lengthened. Further, as treatment time is prolonged, intrafraction motion increases, which in turn increases the need for adaptive replanning, further lengthening treatment time.51 In their trial of MRgRT for patients with thoracic malignancies, Henke et al did not meet their primary endpoint of feasibility, defined as a treatment session lasting less than 80 minutes.13 The authors note they have since improved their institution’s online adaptive process to reduce treatment times. Thus, lengthy treatment times are a limitation new MR-linac users should be aware of and prepared to address.
In addition to the patient inconveniences associated with longer treatment times, lengthier treatments limit the number of patients who can be treated daily on an MR-linac. As a result, patients seen in consultation for MRgRT may have to wait several weeks for availability to start treatment. This limitation can be problematic for patients with fast-growing tumors, tumors in which prolonged treatment time results in inferior outcomes, or in patients on a strict treatment timeline involving a scheduled surgery following radiation.52,53 In addition, given the complexity of this technology and it being relatively nascent, machine downtime is not infrequent, resulting in further delays or the need to transfer patients to standard linacs.54
Several planning limitations associated with MRgRT may further restrict which patients are ideally suited for treatment on these machines. One such limitation is treatment energy. The MR-linac can only deliver 6 to 7 MV treatments, which may not be ideally suited for larger patients undergoing treatment to the abdomen or pelvis. Another limitation is the field size (22 to 24 cm).18,55 Size limitations may preclude the treatment of certain malignancies, such as sarcomas, and make it impractical to treat malignancies requiring coverage of large elective volumes, such as cancers of the head and neck. Since MRgRT treatment can only be done using step-and-shoot static fields, as opposed to VMAT, multiple fields are required to optimize treatment plans. This can be arduous for dosimetrists and physicists to plan and for radiation therapists to deliver, in addition to being lengthy. In their study on use of MRgRT for prostate cancer, for example, Tetar et al found that 15 beams were required to deliver the optimal treatment plan.56 The increased time to deliver a 15-field, step-and-shoot plan can create the very problem an MR-linac is intended to solve, given the increased time for fluctuations in target positioning.
Perhaps the most significant limitation to implementing MRgRT in clinical practice is the heavy resource utilization. Due to complex treatment planning and delivery, which differs significantly from conventional CT-based radiation therapy, a team trained specifically in the plan design and delivery of MR-based radiation therapy is needed.18,57-59 This team involves physicians, radiologists, and physicists.18,57 Henke et al discuss the need to also hire advanced radiation therapists to assist with recontouring to make treatment delivery feasible.13 The time required for personnel to be stationed at MR-linacs during treatment delivery makes them unavailable to perform other duties in the clinic, which can result in serious constraints on physician and physicist staffing. However, not all cases require adaptation on a daily basis.60
It is particularly important for physicians to consider the extended time required at the treatment machine for cases requiring daily plan adaptation. The process for an adaptive case requires an initial isocenter verification check, followed by contouring of the normal organs to review the original plan on the anatomy of the day. This can result in significant time delays given the complexity of defining the interface between normal tissues and target volumes, particularly for abdominal malignancies.61 If an adaptive plan is needed, the physician must remain at the MR-linac while the new plan is run, and then evaluate the new plan to determine acceptability for treatment. In some circumstances, additional iterations of the planning process are required to meet the objectives of the normal tissue constraints and the desired target coverage.47 These situations challenge our physics colleagues, whose expertise is needed to determine how best to adjust the cost functions and which constraints to prioritize. The physician time involved can extend to up to 45 minutes per adaptive plan. After multiple iterations of the planning process, it will then be up to the physician to determine whether gross tumor volume (GTV) coverage should be sacrificed to keep the normal tissue constraints within appropriate limits.
Additional personnel may also be required due to the complexity of this treatment technique. A radiologist with expertise in MRI may need to be recruited to the program to aid the transition of the physician practice to MR-based contouring, which differs substantially from CT-based planning.62,63 For some sites, such as the pancreas, additional fusion of the diagnostic CT scan, which has intravenous and oral contrast, may be necessary to delineate the GTV. For moving targets, such as thoracic and abdominal malignancies, breath-hold techniques may be needed to manage the tumor motion due to respiration. Fusion of the planning MR images with the planning CT images, all obtained with a technique such as deep inspiration breath hold, can pose challenges when these planning images must also be fused with diagnostic images, such as PET (positron emission tomography) and CT images, to verify the target and its position at the time of treatment. Careful attention must be paid to the appearance of the GTV on the MRI images relative to the CT images, because the MR volumes will often appear smaller than on CT.62,63 For sites such as the pancreas, optimizing target delineation is essential to avoid a marginal miss.64
Another consideration for practices newly adopting MRgRT is lack of prospective data to guide use. Several trials are underway investigating the clinical benefits of MRgRT for cancer patients (eg, NCT04075305, NCT04351204). However, limited prospective data are available to indicate which outcomes are improved, and for which cancer patients, relative to treatment on a conventional CT-based system.65 The theoretical benefits of this technology may not translate into real-world benefits.65 Treating patients with a therapy not evaluated in a randomized controlled trial can, in the worst case, be harmful to patients. Clinics with limited resources should consider this lack of data when justifying the resource expenditure for MRgRT.
Future Directions
As MRgRT is increasingly used and newer technology becomes available, many clinical limitations described above may be improved. Improvements in the workflow through automatic reconstruction of the daily delivered dose, implementation of an MRI-only workflow, and the creation of consortiums to allow for a more coordinated, evidence-based introduction of MRgRT into clinical practice, are being explored.66-68 Artificial intelligence is also being explored as a means of automating aspects of treatment planning, such as contouring and plan optimization, to decrease treatment time and resource utilization.69 The need for clinical trials to better address which patients would benefit most from treatment on an MR-linac is also being actively addressed.65 These improvements should eventually allow for more seamless integration of MRgRT into daily clinical practice, reducing the current burden on treatment facilities and patients.
Conclusions
The practical limitations of MRgRT limit the number of cancer patients who would derive the most benefit from this technology. Given the current capabilities of MR-linacs and the limited prospective data, careful patient selection is critical for appropriate resource allocation in practices adopting this technology. Centers seeking to adopt MRgRT into their clinical practices should carefully consider the limitations of this therapy to prepare for its successful implementation.
References
- Mutic S, Dempsey JF. The ViewRay system: magnetic resonance-guided and controlled radiotherapy. Semin Radiat Oncol. 2014;24(3):196-199.
- Raaymakers BW, Jürgenliemk-Schulz IM, Bol GH, et al. First patients treated with a 1.5 T MRI-Linac: clinical proof of concept of a high-precision, high-field MRI guided radiotherapy treatment. Phys Med Biol. 2017;62(23):L41-l50.
- Hall WA, Paulson ES, van der Heide UA, et al. The transformation of radiation oncology using real-time magnetic resonance guidance: a review. Euro J Cancer. 2019;122:42-52.
- Winkel D, Bol GH, Kroon PS, et al. Adaptive radiotherapy: the Elekta Unity MR-linac concept. Clin Transl Radiat Oncol. 2019;18:54-59.
- Noel CE, Parikh PJ, Spencer CR, et al. Comparison of onboard low-field magnetic resonance imaging versus onboard computed tomography for anatomy visualization in radiotherapy. Acta Oncol. 2015;54(9):1474-1482.
- Fischer-Valuck BW, Henke L, Green O, et al. Two-and-a-half-year clinical experience with the world’s first magnetic resonance image guided radiation therapy system. Adv Radiat Oncol. 2017;2(3):485-493.
- El-Bared N, Portelance L, Spieler BO, et al. Dosimetric benefits and practical pitfalls of daily online adaptive MRI-guided stereotactic radiation therapy for pancreatic cancer. Pract Radiat Oncol. 2019;9(1):e46-e54.
- Palacios MA, Bohoudi O, Bruynzeel AME, et al. Role of daily plan adaptation in MR-guided stereotactic ablative radiation therapy for adrenal metastases. Int J Radiat Oncol Biol Phys. 2018;102(2):426-433.
- Tchelebi LT, Zaorsky NG, Rosenberg JC, et al. Reducing the toxicity of radiotherapy for pancreatic cancer with magnetic resonance-guided radiotherapy. Toxicol Sci. 2020.
- Henke L, Kashani R, Robinson C, et al. Phase I trial of stereotactic MR-guided online adaptive radiation therapy (SMART) for the treatment of oligometastatic or unresect
- able primary malignancies of the abdomen. Radiother Oncol. 2018;126(3):519-526.
- Crane CH. Hypofractionated ablative radiotherapy for locally advanced pancreatic cancer. J Radiat Res. 2016;57(S1):i53-i57.
- Hassanzadeh C, Rudra S, Bommireddy A, et al. Ablative five-fraction stereotactic body radiation therapy for inoperable pancreatic cancer using online MR-guided adaptation. Adv Radiat Oncol. 2021;6(1):100506.
- Henke LE, Olsen JR, Contreras JA, et al. Stereotactic MR-guided online adaptive radiation therapy (SMART) for ultracentral thorax malignancies: results of a phase 1 trial. Adv Radiat Oncol. 2018;4(1):201-209.
- Henke L, Kashani R, Robinson C, et al. Phase I trial of stereotactic MR-guided online adaptive radiation therapy (SMART) for the treatment of oligometastatic or unresectable primary malignancies of the abdomen. Radiother Oncol. 2018;126(3):519-526.
- Green OL, Rankine LJ, Cai B, et al. First clinical implementation of real-time, real anatomy tracking and radiation beam control. Med Phys. 2018;45(8):3728-3740.
- Massaccesi M, Cusumano D, Boldrini L, et al. A new frontier of image guidance: organs at risk avoidance with MRI-guided respiratory-gated intensity modulated radiotherapy: Technical note and report of a case. J Appl Clin Med Phys. 2019;20(6):194-198.
- Boldrini L, Cusumano D, Cellini F, Azario L, Mattiucci GC, Valentini V. Online adaptive magnetic resonance guided radiotherapy for pancreatic cancer: state of the art, pearls and pitfalls. Radiat Oncol. 2019;14(1):71.
- Chin S, Eccles CL, McWilliam A, et al. Magnetic resonance-guided radiation therapy: a review. J Med Imag Radiat Oncol. 2020;64(1):163-177.
- Eccles CL, Haider EA, Haider MA, Fung S, Lockwood G, Dawson LA. Change in diffusion weighted MRI during liver cancer radiotherapy: preliminary observations. Acta Oncol. 2009;48(7):1034-1043.
- Weiss E, Ford JC, Olsen KM, et al. Apparent diffusion coefficient (ADC) change on repeated diffusion-weighted magnetic resonance imaging during radiochemotherapy for non-small cell lung cancer: a pilot study. Lung Cancer. 2016;96:113-119.
- King AD, Thoeny HC. Functional MRI for the prediction of treatment response in head and neck squamous cell carcinoma: potential and limitations. Cancer Imag. 2016;16(1):23.
- Foltz WD, Wu A, Chung P, et al. Changes in apparent diffusion coefficient and T2 relaxation during radiotherapy for prostate cancer. J Magn Reson Imag. 2013;37(4):909-916.
- Pasquier D, Hadj Henni A, Escande A, et al. Diffusion weighted MRI as an early predictor of tumor response to hypofractionated stereotactic boost for prostate cancer. Sci Rep. 2018;8(1):10407.
- Tsien C, Cao Y, Chenevert T. Clinical applications for diffusion magnetic resonance imaging in radiotherapy. Semin Radiat Oncol. 2014;24(3):218-226.
- Cao Y. The promise of dynamic contrast-enhanced imaging in radiation therapy. Semin Radiat Oncol. 2011;21(2):147-156.
- Boldrini L, Cusumano D, Chiloiro G, et al. Delta radiomics for rectal cancer response prediction with hybrid 0.35 T magnetic resonance-guided radiotherapy (MRgRT): a hypothesis-generating study for an innovative personalized medicine approach. Radiol Med. 2019;124(2):145-153.
- Tchelebi LT, Romesser PB, Feuerlein S, et al. Magnetic resonance guided radiotherapy for rectal cancer: expanding opportunities for non-operative management. Cancer Control. 2020;27(1):1073274820969449.
- Yang Y, Cao M, Sheng K, et al. Longitudinal diffusion MRI for treatment response assessment: preliminary experience using an MRI-guided tri-cobalt 60 radiotherapy system. Med Phys. 2016;43(3):1369-1373.
- Kooreman ES, van Houdt PJ, Nowee ME, et al. Feasibility and accuracy of quantitative imaging on a 1.5 T MR-linear accelerator. Radiother Oncol. 2019;133:156-162.
- van Houdt PJ, Yang Y, van der Heide UA. Quantitative magnetic resonance imaging for biological image-guided adaptive radiotherapy. Front Oncol. 2021;10(3190).
- Corradini S, Alongi F, Andratschke N, et al. MR-guidance in clinical reality: current treatment challenges and future perspectives. Radiat Oncol. 2019;14(1):92.
- Groot Koerkamp ML, Vasmel JE, Russell NS, et al. Optimizing MR-guided radiotherapy for breast cancer patients. Front Oncol. 2020;10(1107).
- Crockett CB, Samson P, Chuter R, et al. Initial clinical experience of MR-guided radiotherapy for non-small cell lung cancer. Front Oncol. 2021;11(157).
- Boldrini L, Intven M, Bassetti M, Valentini V, Gani C. MR-guided radiotherapy for rectal cancer: current perspective on organ preservation. Front Oncol. 2021;11(530).
- Intven MPW, de Mol van Otterloo SR, Mook S, et al. Online adaptive MR-guided radiotherapy for rectal cancer; feasibility of the workflow on a 1.5T MR-linac: clinical implementation and initial experience. Radiother Oncol. 2021;154:172-178.
- Chiloiro G, Boldrini L, Meldolesi E, et al. MR-guided radiotherapy in rectal cancer: first clinical experience of an innovative technology. Clin Transl Radiat Oncol. 2019;18:80-86.
- Tocco BR, Kishan AU, Ma TM, Kerkmeijer LGW, Tree AC. MR-guided radiotherapy for prostate cancer. Front Oncol. 2020;10:616291-616291.
- Bruynzeel AME, Tetar SU, Oei SS, et al. A prospective single-arm phase II study of stereotactic magnetic-resonance-guided adaptive radiotherapy for prostate cancer: early toxicity results. Int J Radiat Oncol Biol Phys. 2019.
- Luterstein E, Cao M, Lamb JM, et al. Clinical outcomes using magnetic resonance–guided stereotactic body radiation therapy in patients with locally advanced cholangiocarcinoma. Adv Radiat Oncol. 2020;5(2):189-195.
- Klüter S, Katayama S, Spindeldreier CK, et al. First prospective clinical evaluation of feasibility and patient acceptance of magnetic resonance-guided radiotherapy in Germany. Strahlenther Onkol. 2020;196(8):691-698.
- Hal WA, Straza MW, Chen X, et al. Initial clinical experience of stereotactic body radiation therapy (SBRT) for liver metastases, primary liver malignancy, and pancreatic cancer with 4D-MRI based online adaptation and real-time MRI monitoring using a 1.5 Tesla MR-linac. PLOS ONE. 2020;15(8):e0236570.
- Bohoudi O, Bruynzeel AME, Senan S, et al. Fast and robust online adaptive planning in stereotactic MR-guided adaptive radiation therapy (SMART) for pancreatic cancer. Radiother Oncol. 2017;125(3):439-444.
- Feldman AM, Modh A, Glide-Hurst C, Chetty IJ, Movsas B. Real-time magnetic resonance-guided liver stereotactic body radiation therapy: an institutional report using a magnetic resonance-linac system. Cureus. 2019;11(9):e5774.
- Rosenberg SA, Henke LE, Shaverdian N, et al. A multi-institutional experience of MR-guided liver stereotactic body radiation therapy. Adv Radiat Oncol. 2019;4(1):142-149.
- Rudra S, Jiang N, Rosenberg SA, et al. Using adaptive magnetic resonance image-guided radiation therapy for treatment of inoperable pancreatic cancer. Cancer Med. 2019;8(5):2123-2132.
- Chuong MD, Springett GM, Freilich JM, et al. Stereotactic body radiation therapy for locally advanced and borderline resectable pancreatic cancer is effective and well tolerated. Int J Radiat Oncol Biol Phys. 2013;86(3):516-522.
- Hall WA, Small C, Paulson E, et al. Magnetic resonance guided radiation therapy for pancreatic adenocarcinoma, advantages, challenges, current approaches, and future directions. Front Oncol. 2021;11(867).
- Boldrini L, Corradini S, Gani C, et al. MR-guided radiotherapy for liver malignancies. Front Oncol. 2021;11(1053).
- Hehakaya C, Van der Voort van Zyp JR, Lagendijk JJW, Grobbee DE, Verkooijen HM, Moors EHM. Problems and promises of introducing the magnetic resonance imaging linear accelerator into routine care: the case of prostate cancer. Front Oncol. 2020;10(1741).
- Ding S, Li Y, Liu H, et al. Comparison of intensity modulated radiotherapy treatment plans between 1.5T MR-linac and conventional linac. Technol Cancer Res Treat. 2021;20:1533033820985871-1533033820985871.
- Sevak PR, Nejad-Davarani S, Kim J, Weiss S, Elshaikh MA, Glide-Hurst CK. Intrafractional motion of target volumes and organs at risk due to bladder filling: implications for MR-only prostate radiation therapy. Int J Radiat Oncol Biol Phys. 2017;99(2):E720-E721.
- Perez RO, Habr-Gama A, Gama-Rodrigues J, et al. Accuracy of positron emission tomography/computed tomography and clinical assessment in the detection of complete rectal tumor regression after neoadjuvant chemoradiation: long-term results of a prospective trial (National Clinical Trial 00254683). Cancer. 2012;118(14):3501-3511.
- Shaikh T, Handorf EA, Murphy CT, Mehra R, Ridge JA, Galloway TJ. The impact of radiation treatment time on survival in patients with head and neck cancer. Int J Radiat Oncol Biol Phys. 2016;96(5):967-975.
- Keller BM, Campbell M, Ruschin ME, et al. MR-linac radiotherapy in year one: experience’s in imaging, patient treatment and data collection. Int J Radiat Oncol Biol Phys. 2020;108(3):e249-e250.
- Chuter RW, Whitehurst P, Choudhury A, van Herk M, McWilliam A. Technical note: investigating the impact of field size on patient selection for the 1.5T MR-linac. Med Phys. 2017;44(11):5667-5671.
- Tetar SU, Bruynzeel AME, Lagerwaard FJ, Slotman BJ, Bohoudi O, Palacios MA. Clinical implementation of magnetic resonance imaging guided adaptive radiotherapy for localized prostate cancer. Phys Imag Radiat Oncol. 2019;9:69-76.
- van Herk M, McWilliam A, Dubec M, Faivre-Finn C, Choudhury A. Magnetic resonance imaging-guided radiation therapy: a short strengths, weaknesses, opportunities, and threats analysis. Int J Radiat Oncol Biol Phys. 2018;101(5):1057-1060.
- Murray J, Tree AC. Prostate cancer – advantages and disadvantages of MR-guided RT. Clin Transl Radiat Oncol. 2019;18:68-73.
- Eccles CL, Campbell M. Keeping up with the hybrid magnetic resonance linear accelerators: how do radiation therapists stay current in the era of hybrid technologies? J Med Imaging Radiat Sci. 2019;50(2):195-198.
- Sandoval ML, Youssef I, Latifi K, et al. Non-adaptive MR-Guided radiotherapy for prostate sbrt: less time, equal results. J Clin Med. 2021;10(15):3396.
- Tyran M, Jiang N, Cao M, et al. Retrospective evaluation of decision-making for pancreatic stereotactic MR-guided adaptive radiotherapy. Radiother Oncol. 2018;129(2):319-325.
- Hall WA, Heerkens HD, Paulson ES, et al. Pancreatic gross tumor volume contouring on computed tomography (CT) compared with magnetic resonance imaging (MRI): results of an international contouring conference. Pract Radiat Oncol. 2018;8(2):107-115.
- Heerkens HD, Hall WA, Li XA, et al. Recommendations for MRI-based contouring of gross tumor volume and organs at risk for radiation therapy of pancreatic cancer. Pract Radiat Oncol. 2017;7(2):126-136.
- Barrord M, Ahmad S, Patel S, et al. Patterns of failure after neoadjuvant stereotactic body radiation therapy or fractionated chemoradiation in resectable and borderline resectable pancreatic cancer. Pancreas. 2020;49(7):941-946.
- Verkooijen HM, Henke LE. Sensible introduction of MR-guided radiotherapy: a warm plea for the RCT. Front Oncol. 2021;11(872).
- Menten MJ, Mohajer JK, Nilawar R, et al. Automatic reconstruction of the delivered dose of the day using MR-linac treatment log files and online MR imaging. Radiother Oncol. 2020;145:88-94.
- Johnstone E, Wyatt JJ, Henry AM, et al. Systematic review of synthetic computed tomography generation methodologies for use in magnetic resonance imaging-only radiation therapy. Int J Radiat Oncol Biol Phys. 2018;100(1):199-217.
- Verkooijen HM, Kerkmeijer LGW, Fuller CD, et al. R-IDEAL: a framework for systematic clinical evaluation of technical innovations in radiation oncology. Front Oncol. 2017;7:59.
- Wang C, Zhu X, Hong JC, Zheng D. Artificial intelligence in radiotherapy treatment planning: present and future. Technol Cancer Res Treat. 2019;18:1533033819873922.
Citation
Tchelebi LT, Zaorsky NG, Rosenberg J, Latifi K, Hoffe H . Integrating MR-Guided Radiation Therapy Into Clinical Practice: Clinical Advantages and Practical Limitations. Appl Radiat Oncol. 2021;(3):16-23.
October 5, 2021