Noninvasive Cardiac Radioablation at Washington University: Past, Present, Future Directions for the Treatment of VT
Images
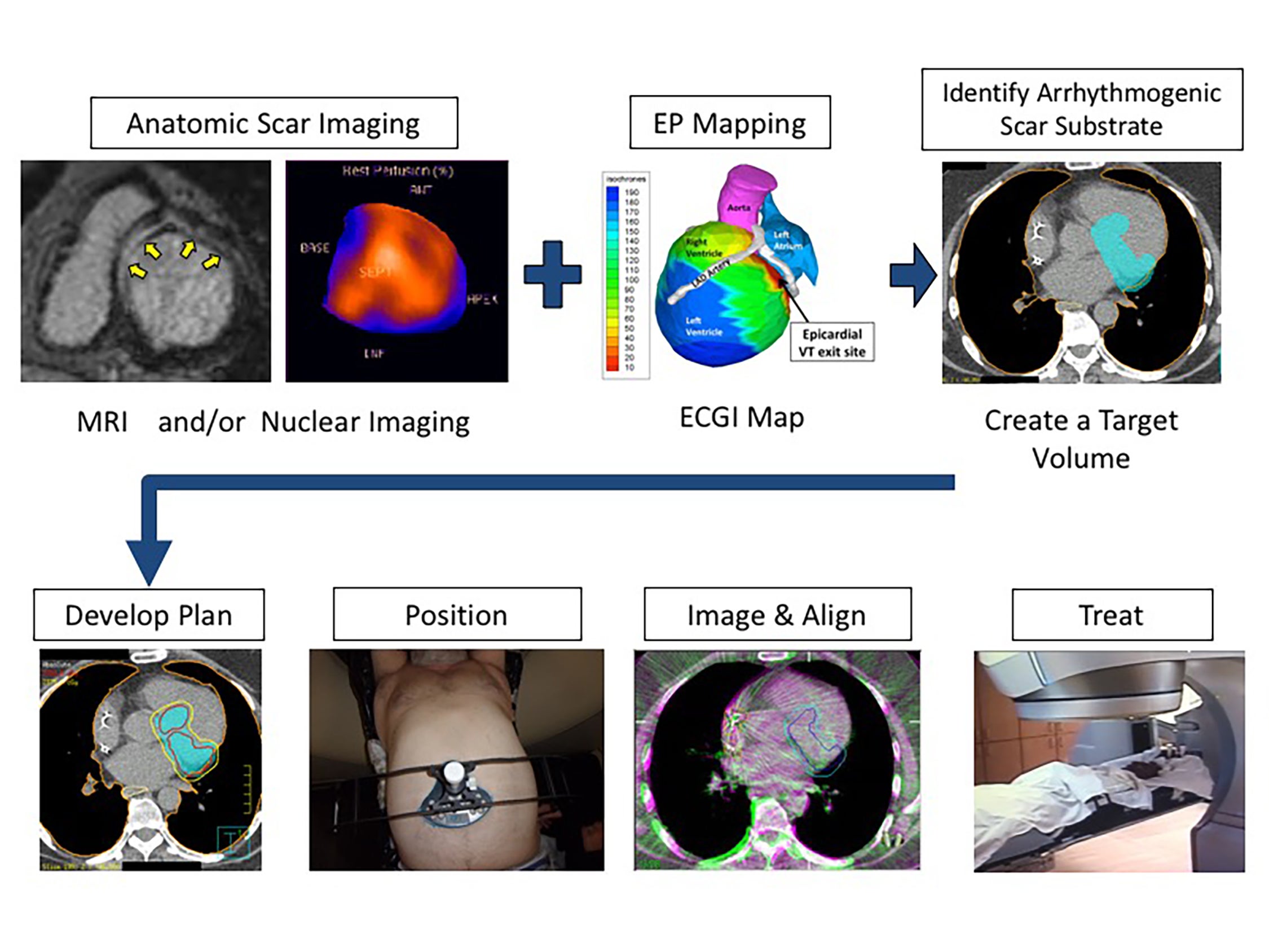
SA-CME credits are available for this article here.
Among the many types of cardiac arrhythmias, ventricular tachycardia (VT) represents a potentially fatal arrhythmia in the setting of abnormal anatomical and physiological pathways of the heart. Specifically, these aberrant pathways result from cardiac injury to the ventricles, either by ischemic causes (postmyocardial infarction) or nonischemic causes (inflammatory or secondary to other systemic pathologies). Regardless of the underlying etiology, these arrhythmias are associated with a high mortality rate. Gold standard therapies for VT include catheter ablation and medical therapies. Catheter ablation is a procedure performed over several hours under anesthesia in which vascular access is obtained, the location of the ventricular arrhythmia is mapped using the catheter, and electrical tracts are ablated using thermal ablation, essentially converting a functional scar into a nonfunctional scar. Anti-arrhythmic drugs generally work by affecting the repolarization phases of the heart through sodium, potassium and/or calcium channels to slow the conduction rate. While these are effective for many patients with VT, both approaches can be associated with significant toxicities. For example, a recent national retrospective analysis including more than 4600 patients receiving catheter ablation for ischemic VT showed the overall rate of any in-hospital complication was 11% with an in-hospital mortality of 1.6%.1 The cornerstone of medical management has been amiodarone, which unfortunately can cause pulmonary fibrosis, hypothyroidism, and other systemic effects. Even for patients who can tolerate procedural and medical management, these therapies are not always durable and can result in treatment refractory VT.2-4 For patients with refractory disease, one series exploring noncatheter ablation salvage such as transcoronary alcohol ablation, epicardial window, and cryoablation demonstrated a 25% complication rate and 10% 30-day mortality.5
In a similar lineage to other applications of stereotactic body radiation therapy (SBRT), noninvasive cardiac radioablation has emerged as a potential salvage treatment option for patients who have either failed procedural or medical management, or have contraindications to receive those therapies again. The recently published phase I/II Electrophysiology-Guided (EP) Noninvasive Cardiac Radioablation for Ventricular Tachycardia trial (ENCORE-VT, NCT02919618) demonstrated early safety and efficacy of this novel use of SBRT.6 In this review, we will discuss the lessons learned from ENCORE-VT, our current use and workflow for treatment refractory ventricular tachycardia patients, and future directions for this treatment modality.
Development of Noninvasive Cardiac Radioablation
Prior to implementing noninvasive cardiac radioablation in humans, preclinical studies laid the groundwork for this treatment approach.7-10 Previous work by Zei and colleagues demonstrated the effectiveness of using SBRT to target the superior pulmonary veins (as done in atrial fibrillation ablative procedures) with 25 Gy.11 Similarly, Packer and colleagues demonstrated the effectiveness of creating a complete atrioventricular nodal block using 25 Gy in a single fraction in a porcine model.12 Additionally, these animal models were monitored for 3 to 6 months following the procedure, with no evidence of collateral injury found at the time of histopathological assessment at study conclusion. Of note, these preclinical studies were done in animal models with structurally normal hearts and were found to create fibrotic changes in the distribution of the target volume, over a period of months from the time of treatment. This mechanism of ablation is fundamentally different from catheter-based approaches, which use radiofrequency energy to cauterize the arrhythmogenic circuit, over the period of days to weeks.13 However, no preclinical studies to date have examined electrophysiologic and histologic changes in models with aberrant conduction pathways due to intrinsic cardiac disease and, therefore, it is unknown how the latent period and tissue changes may differ from healthy models.
While these studies demonstrated the feasibility of cardiac radioablation to alter electrical conduction, use in humans was limited because the arrhythmogenic pathways in the ventricular muscle needed to be mapped so a treatment volume could be appropriately delineated. With conventional catheter ablation, the mapping and treatment are done as one procedure, as the catheter can be used to both map the arrhythmogenic circuit and then provide ablation to that delineated area. Therefore, the attractiveness of a noninvasive cardiac radioablation option was significantly limited as the patient still needed a catheter-based mapping procedure. If the catheter is already placed directly at the target, one can see the willingness to ablate (or re-ablate), vs ending the procedure without direct treatment at that time. While conventional imaging tests such as cardiac MRI, single photon emission computed tomography (SPECT), positron emission tomography (PET), and echocardiogram can provide some gross/anatomical features of areas of fibrosis in which the arrhythmia likely originates, it gives us no electrical information to delineate the actual pathway of the arrhythmia. One development able to bridge anatomical information with aberrant electrical pathways normally obtained by invasive catheter mapping is electrocardiographic imaging (ECGI).14,15 During this procedure, the patient wears a vest containing 256 electrodes that individually capture electrocardiograms based on their distance and orientation from the heart. While still wearing the vest, the patient also undergoes a CT scan to generate anatomical coordinates for each of the electrodes in relation to the heart. With the anatomical and electrical mapping, an algorithm is used to translate the body surface potentials back to the electrical potentials on the epicardial surface.16 This technology has now been formally developed as the CardioInsight Noninvasive 3D Mapping System (Medtronic).
With the integration of electrocardiographic imaging to identify the VT substrate externally, the stage was then set for an entirely noninvasive cardiac radioablation workflow. On the ENCORE-VT phase I/II trial, we elected to deliver linac-based SBRT (Edge, Varian) as this would allow us to take advantage of a well-established thoracic workflow in terms of motion control, treatment planning, on-board fluoroscopy, and minimization of neutron exposure for implantable cardioverter defibrillators (ICDs). This workflow and dosimetric objectives were recently described by Knutson et al.17 Briefly, the treatment target (here, a gross target volume [GTV]), is compiled upon review of all available radiologic imaging and electrophysiologic data including 12-lead ECG and ECGI. A respiratory correlated 4-dimensional CT (4D-CT) with abdominal compression is acquired and co-registered to a free-breathing CT to assess target motion. Recently, we upgraded our CT simulator to perform a respiratory 4D-CT with ECG-gated imaging to assess cardiac motion (Siemens). With this summation of respiratory and cardiac movement, an internal target volume (ITV) is then generated. Of note, ITV delineation for cardiac radioablation planning presents a new challenge for radiation oncologists who may be used to contouring based on excursion of a mass. For this, we have noticed that superimposing a 5-mm grid directly onto the CT images during ITV delineation helps document the extent of target movement and provide appropriate coverage boundaries. Initially, a 5-mm expansion was done to create the planning target volume (PTV) from the ITV, although with additional experience we have now decreased our PTV margin to 2 to 3 mm. A dose of 25 Gy in a single fraction is then delivered (with allowance of up to a 35 Gy hotspot within the ITV). Our current institutional workflow for noninvasive cardiac radioablation is shown in Figure 1.
In July 2016, ENCORE-VT was opened to evaluate safety and efficacy of noninvasive cardiac radioablation in patients with refractory VT, defined as failure of ≥ 1 antiarrhythmic medications and ≥ 1 catheter ablation or having a contraindication to catheter ablation.6 The primary safety endpoint was defined as the rate of ≤ 90-day serious adverse events (SAE, ≥ grade 3) and the primary efficacy endpoint was defined as the number of subjects with any reduction in the number of ICD therapies, including shocks and/or antitachycadia pacing (ATP). The results of this clinical trial demonstrated low risk of SAEs, significantly decreased VT episodes in 95% of patients, decreased use of amiodarone and dual-antiarrhythmic medications, and significant improvements in quality-of-life metrics.
Current Use of Noninvasive Cardiac Radioablation for Refractory Ventricular Tachycardia
A substantial clinical need for patients with refractory VT has led to continued off-label use of noninvasive cardiac radioablation in select patients as we await opening of the next prospective trial. As of February 2020, we have now treated 39 patients. As more patients are treated and our follow-up becomes longer, our focus is shifting to continued improvements in workflow, close monitoring for long-term toxicities, and collaboration with other centers planning to use noninvasive cardiac radioablation.
In examining our treatment planning patterns over time, we have seen evidence of a learning curve during the course of ENCORE-VT. Specifically, we saw with the more recent cohort of patients a significant decrease in PTV volume, which was reflective of smaller GTV volumes as there was no changes in motion management or PTV margins during the clinical trial.17 Subjective decreases in the R50 (ratio of the volume of the 12.5 Gy isodose to the PTV), gradient measure (average distance between the 12.5 Gy equivalent spherical volume and the 25 Gy equivalent spherical volume), homogeneity index (ratio of the maximum dose to the prescription dose), and treatment time were also observed in this small group of patients (n = 16), but this did not reach significance. These findings have helped establish a new range of objectives in treatment planning and evaluation that are now implemented in our clinic. For example, examining the clinical outcomes and target volume size, we saw that no patients with a PTV > 200 cc lived to 1 year.18 An association between SAEs and PTVs was not found, suggesting that the mortality rate with large volumes was more likely a surrogate for more severe heart disease/dysfunction rather than cardiac toxicity from radiation therapy. However, this value now serves as a possible prognostic factor for early mortality.
As our follow-up of patients participating in ENCORE-VT lengthens, we continue to become aware of possible short- and long-term toxicities. In the longer-term follow-up presented at ASTRO 2019, we have now described 2 cases of late-grade 3 pericarditis (both occurring > 2 years from the time of radiation, treated with steroids) and 1 patient with a grade 4 gastropericardial fistula (2.4 years after radiation) requiring surgical intervention.19 In reviewing the plan of the patient who developed the gastropericardial fistula, we did see that the target was in the apex of the left ventricle – the portion of the heart closest to the anterior left diaphragm and stomach. Currently, any targets including the left ventricular apex are now planned to optimize on the stomach, and the patient is consented regarding the risk of gastric ulceration and fistula. Diligent clinical follow-up for these patients will be crucial in the years to come. From the ENCORE-VT trial, we documented that the left anterior descending artery (LAD), which is the major blood supply to the left ventricle for the majority of the population, received a median dose of 10 Gy (IQR 10.7, EQD2 26.4 Gy). Similarly, the left circumflex artery received a median dose of 9.2 Gy (IQR 6.3, EQD2 22.4 Gy).17 While we have yet to see any direct evidence of radiation-related coronary adverse events among treated patients, these late effects could take years to develop. This will be crucial to document and understand as durable control of ventricular arrhythmias improves survival to a point that radiation late effects must be considered.
With this demonstration of clinical efficacy for salvage therapy, international interest in this therapy continues to develop. To date, the recently formed Center for Noninvasive Radioablation (CNCR, pronounced “Conquer”) at our institution has collaborated with 27 domestic and international hospitals to remotely review patient cases, target mapping, and treatment planning. Similarly, other groups have initiated collaborative work to treat and manage patients receiving noninvasive cardiac radioablation such as the Standardized Treatment and Outcome Platform for Stereotactic Therapy of Re-entrant Tachycardia by a Multidisciplinary Consortium (StopStorm), representing the efforts of radiation oncologists, electrophysiologists and physicists from 7 European countries.
Noninvasive Cardiac Radioablation: Going Forward
The future of noninvasive cardiac radioablation involves many potential avenues for research: elucidation of the mechanisms of radiobiologic effectiveness, continued refinement of target delineation, dose finding studies, diligent monitoring for late toxicities, and scalability of this workflow to centers regardless of geography. Ultimately, multi-institutional phase II and III trials to compare noninvasive cardiac radioablation against repeat catheter ablation for refractory ventricular tachycardia will help further delineate the role of this therapy. These trials will only be possible through continued collaboration on both the institutional level (electrophysiology, radiation oncology and medical physics) and through cooperative groups with specific end-to-end testing metrics, quality control, and central plan review. Another question that will be addressed in the future is understanding the relative benefits and risks of various treatment modalities that could deliver this modality. While intensity-modulated proton therapy (IMPT) could offer dosimetric advantages to organs at risk including nontarget heart tissue, potential issues include neutron scatter that could damage ICDs/pacemakers in patients who depend on these devices, significant sensitivity to motion, and changes in volume status of the patient from the time of simulation to the time of treatment. In ENCORE-VT, most patients had class III or IV heart failure, and as such may have had significant changes in weight and cardiac filling from week to week. Another challenge to proton therapy would be confirming target localization on the day of treatment — in our current workflow process we use both a cone-beam CT and fluoroscopy to adjust positioning immediately prior to treatment if required. It is also possible that intrafraction cone-beam imaging could be of benefit in complex anatomical targets and/or targets immediately adjacent to the esophagus or diaphragm/stomach.
Another treatment approach could involve MR-guidance, which would offer the benefit of real-time imaging during treatment. However, while a benefit of MR-guided therapy in cancer therapy is the ability to gate tumor targets relative to surrounding soft tissue through continuous real-time imaging, this has not yet been shown to apply to cardiac targets as there is no discernable difference between the treatment volume and the nontarget heart tissue. Therefore, gating would have to occur based on the entire heart or a surrogate structure in the thorax, which may not offer a clear benefit compared to a standard ITV approach. However, we have described the use of MR-guided therapy for a case in which VT was being caused by a cardiac fibroma where the mass was visible and able to be tracked.20
Conclusions
Similar to SBRT use for other malignancies, early clinical trial work has shown that noninvasive cardiac radioablation for VT is a potentially safe, effective, and durable salvage treatment for a patient population that has exhausted procedural and medical management options. However, understanding the threshold of potential benefit and long-term risk of toxicities will be crucial in delineating the patient population that should receive this treatment. Future endeavors in radiobiology mechanisms, clinical trial development, and quality improvement will facilitate the continued development of this new application of SBRT.
References
- Palaniswamy C, Kolte D, Harikrishnan P, et al. Catheter ablation of postinfarction ventricular tachycardia: ten-year trends in utilization, in-hospital complications, and in-hospital mortality in the United States. Hear Rhythm. 2014;11(11):2056-2063. doi:10.1016/j.hrthm.2014.07.012
- Wolf M, Sacher F, Cochet H, et al. Long-term outcome of substrate modification in ablation of post-myocardial infarction ventricular tachycardia. Circ Arrhythm Electrophysiol. 2018;11(2):e005635. doi:10.1161/CIRCEP.117.005635
- Yokokawa M, Desjardins B, Crawford T, Good E, Morady F, Bogun F. Reasons for recurrent ventricular tachycardia after catheter ablation of post-infarction ventricular tachycardia. J Am Coll Cardiol. 2013;61(1):66-73. doi:10.1016/j.jacc.2012.07.059
- Kumar S, Romero J, Mehta NK, et al. Long-term outcomes after catheter ablation of ventricular tachycardia in patients with and without structural heart disease. Heart Rhythm. 2016;13(10):1957-1963. doi:10.1016/j.hrthm.2016.07.001
- Kumar S, Barbhaiya CR, Sobieszczyk P, et al. Role of alternative interventional procedures when endo- and epicardial catheter ablation attempts for ventricular arrhythmias fail. Circ Arrhythmia Electrophysiol. 2015;8(3):606-615. doi:10.1161/CIRCEP.114.002522
- Robinson CG, Samson PP, Moore KMS, et al. Phase I/II trial of Electrophysiology-Guided Noninvasive Cardiac Radioablation for Ventricular Tachycardia. Circulation. 2019;139(3):313-321. doi:10.1161/CIRCULATIONAHA.118.038261
- Zei PC, Wong D, Gardner E, Fogarty T, Maguire P. Safety and efficacy of stereotactic radioablation targeting pulmonary vein tissues in an experimental model. Heart Rhythm. 2018;15(9):1420-1427. doi:10.1016/j.hrthm.2018.04.015
- Sharma A, Wong D, Weidlich G, et al. Noninvasive stereotactic radiosurgery (CyberHeart) for creation of ablation lesions in the atrium. Heart Rhythm. 2010;7(6):802-810. doi:10.1016/j.hrthm.2010.02.010
- Lehmann HI, Richter D, Prokesch H, et al. Atrioventricular node ablation in Langendorff-perfused porcine hearts using carbon ion particle therapy: methods and an in vivo feasibility investigation for catheter-free ablation of cardiac arrhythmias. Circ Arrhythm Electrophysiol. 2015;8(2):429-438. doi:10.1161/CIRCEP.114.002436
- Lehmann HI, Graeff C, Simoniello P, et al. Feasibility study on cardiac arrhythmia ablation using high-energy heavy ion beams. Sci Rep. 2016;6:38895. doi:10.1038/srep38895
- Zei PC, Wong D, Gardner E, Fogarty T, Maguire P. Safety and efficacy of stereotactic radioablation targeting pulmonary vein tissues in an experimental model. Heart Rhythm. 2018;15(9):1420-1427. doi:10.1016/j.hrthm. 2018.04.015
- Lehmann HI, Deisher AJ, Takami M, et al. External arrhythmia ablation using photon beams. Circ Arrhythmia Electrophysiol. 2017;10(4):1-8. doi:10.1161/CIRCEP.116.004304
- Tung R, Boyle NG, Shivkumar K. Catheter ablation of ventricular tachycardia. Circulation. 2010;122(3):e389-391. doi:10.1161/CIRCULATIONAHA.110.963371
- Wang Y, Cuculich PS, Zhang J, et al. Noninvasive electroanatomic mapping of human ventricular arrhythmias with electrocardiographic imaging (ECGI). Sci Transl Med. 2011;3(98):98ra84. doi:10.1126/scitranslmed.3002152
- Ghanem RN, Jia P, Ramanathan C, Ryu K, Markowitz A, Rudy Y. Noninvasive electrocardiographic imaging (ECGI): comparison to intraoperative mapping in patients. Heart Rhythm. 2005;2(4):339-354. doi:10.1016/j.hrthm.2004.12.022
- Cheniti G, Puyo S, Martin CA, et al. Noninvasive mapping and electrocardiographic imaging in atrial and ventricular arrhythmias (CardioInsight). Card Electrophysiol Clin. 2019;11(3):459-471. doi:10.1016/j.ccep.2019.05.004
- Knutson NC, Samson PP, Hugo GD, et al. Radiation therapy workflow and dosimetric analysis from a phase 1/2 trial of noninvasive cardiac radioablation for ventricular tachycardia. Int J Radiat Oncol Biol Phys. 2019;104(5):1114-1123. doi:10.1016/j.ijrobp.2019.04.005
- Samson PP. S-PO01-001 to S-PO01-175. Heart Rhythm. 2019;16(5):S93-S141. doi:10.1016/j.hrthm.2019.04.014
- Robinson CG, Samson P, Moore KMS, et al. Longer term results from a phase I/II Study of EP-guided Noninvasive Cardiac Radioablation for Treatment of Ventricular Tachycardia (ENCORE-VT). Int J Radiat Oncol Biol Phys. 2019;105(3):682. doi:10.1016/j.ijrobp.2019.08.032
- Gach HM, Green OL, Cuculich PS, et al. Lessons learned from the first human low-field MRI guided radiation therapy of the heart in the presence of an implantable cardiac defibrillator. Pract Radiat Oncol. 2019;9(4):274-279. doi:10.1016/j.prro.2019.02.003
Citation
Samson P, Hugo G, Moore K, Knutson N, Cuculich P, Robinson C. Noninvasive Cardiac Radioablation at Washington University: Past, Present, Future Directions for the Treatment of VT. Appl Radiat Oncol. 2020;(1):10-14.
April 4, 2020