Review ArticlePediatric Cancer
Pediatric proton therapy in 2015: Indications, applications and considerations
Images
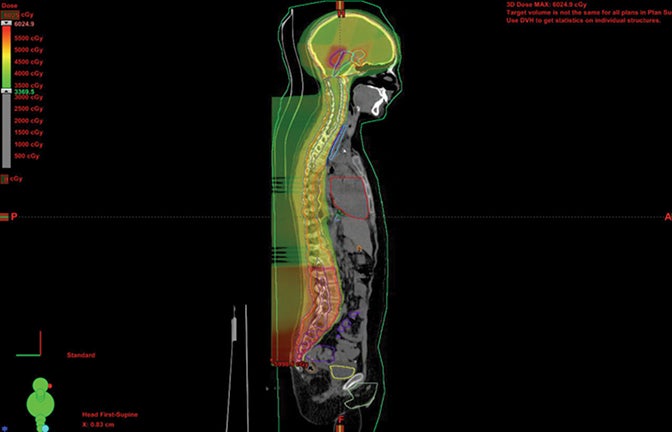
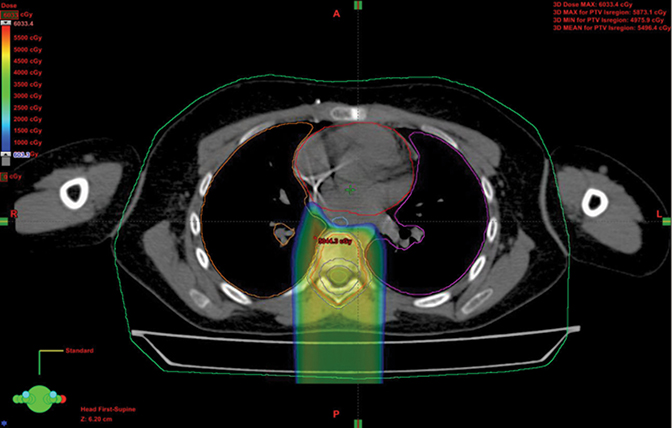
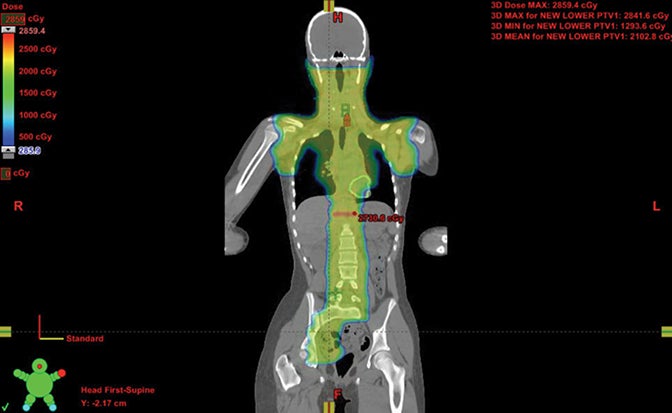
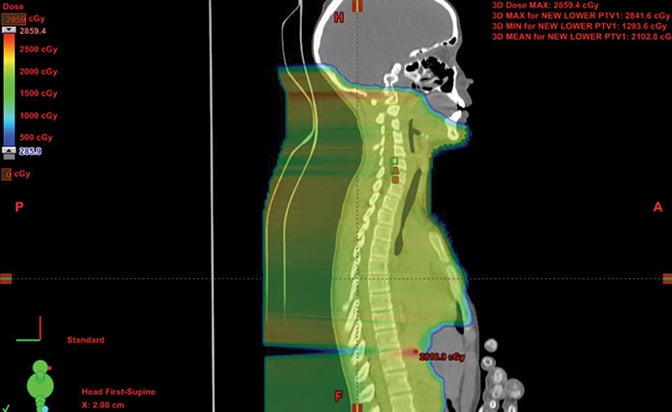
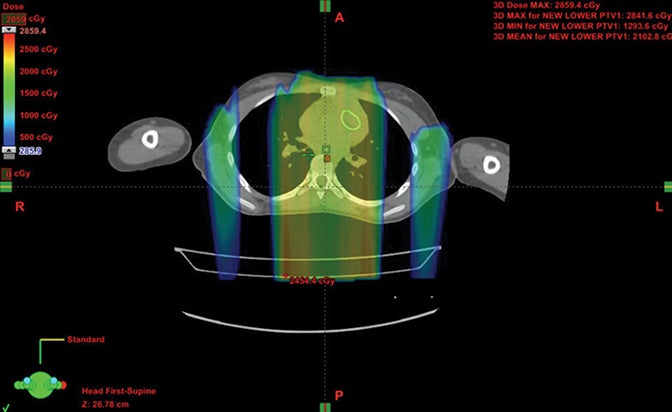
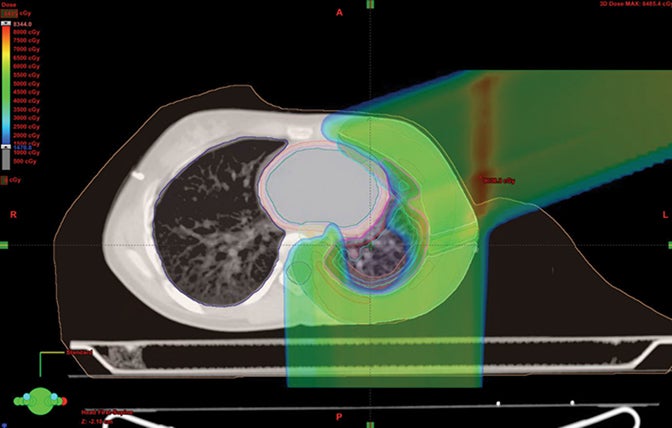
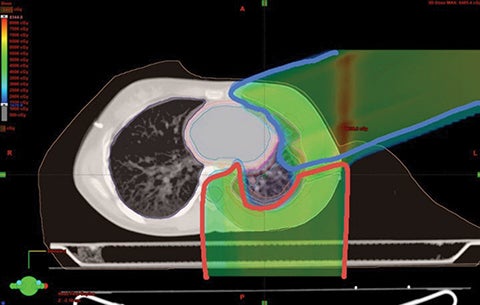
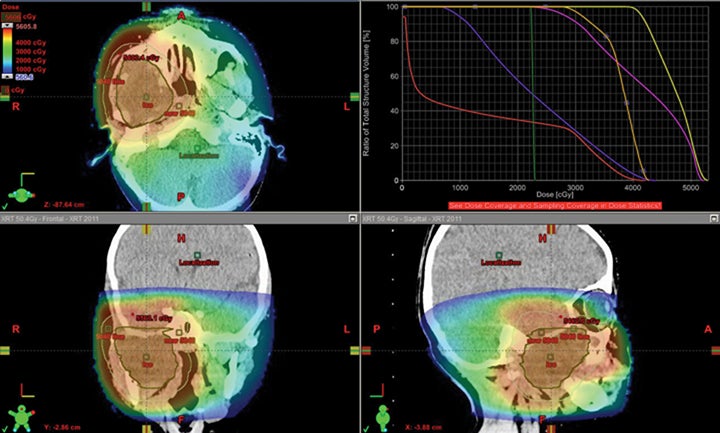
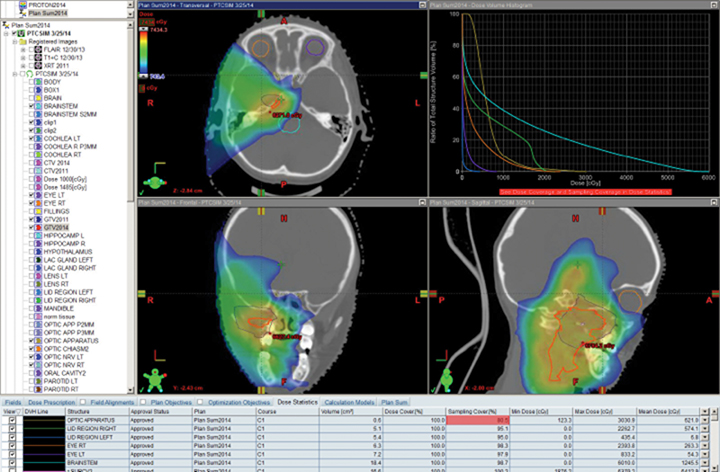
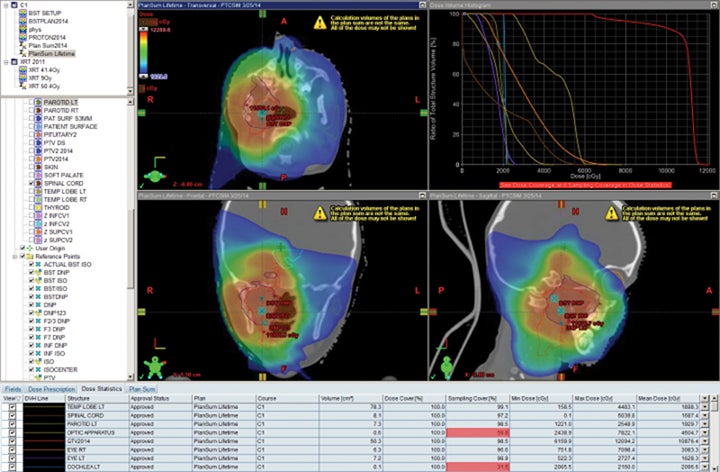
Proton therapy is a complex and important tool in treating cancer. It is not the only form of radiation therapy to use with children, nor is it always the best. However, in the right setting it offers significant advantages over other forms of radiation therapy, and should be considered the optimal choice for treating pediatric patients in those settings.
The first thought that comes to mind with proton therapy is pediatric cancer, especially for our youngest patients.1 This is not a random thought; it is based on integral dose advantages protons have over standard photon radiation therapy when treating children.2 This brief review of pediatric proton radiotherapy discusses the clear indications and contraindications of proton therapy in this population, how the latter may shift to the former, concepts and epidemiological data to support practice patterns, and problems and controversies when caring for pediatric cancer patients.3,4
Next to curing the patient, the primary goal of pediatric radiation oncologists is avoiding late effects. In fact, both goals are nearly equal, as a cured child saddled with debilitating late effects that are preventable is an unacceptable outcome. To prevent late effects, radiation oncologists use the following: radiation avoidance, the lowest dose possible without causing loss of disease control, and dose modulation to avoid organs at risk (OARs). Use of protons is one method of modulating dose to avoid OARs when the dose is high and OARs are close. In adults, intensity-modulated radiation therapy (IMRT) may be ideal, but pediatric radiation oncologists must consider the whole body and OAR needs (beyond those typical for adults), with integral dose minimization a major goal. Models for second cancer development exist that strongly support proton therapy use to optimize normal tissue avoidance.4-7 In one paper, the risk of developing a secondary cancer from craniospinal irradiation (CSI) in a young child is estimated at < 10% lifetime with passive scattered protons, and > 90% with photons.4
Strong indications for proton therapy
CSI
Patients with diseases requiring CSI are a clear-cut population benefitting from proton therapy. The benefit comes from 2 factors: 1) avoidance of anterior organs such as the heart, lungs, bowel, liver, esophagus, thyroid, and bladder; and 2) in addition to the primary fields, more conformal boosts allow better avoidance of critical brain structures such as the hippocampi and cochleae. In fully grown children, bone marrow dose can be minimized by sparing the anterior portion of the vertebral body. The latter advantage decreases with the total overall CSI dose, but is still important.8,9 Figure 1 demonstrates supine CSI in an awake patient.
In atypical cases such as high-grade glioma and sarcoma (as in Figure 1), the doses one can achieve via proton beam typically treat less normal tissue and can avoid internal organs better than other forms of external-beam radiation therapy. For example, at the Indiana University Health Proton Therapy Center (IUHPTC), all forms of medulloblastoma were offered proton therapy, and other types of tumors adjacent to the spinal cord received high doses with protons, with no nausea induction or decreased blood counts in patients.10 In rare cases, retreatment of spine lesions is possible to doses > 100 Gy with proton therapy with spinal-cord sparing; other forms of therapy would likely exceed OAR tolerance. Like photons, proton CSI is delivered with the patient supine or prone, using general anesthesia as needed.11-12 And like photons, but to a much lesser extent, protons deliver small doses to adjacent tissue.13-15
Intracranial and base-of-skull tumors
Tumors of the brain, calvarium, and base of skull are often best treated with proton therapy, because the more we study the brain in terms of late effects, the more we find that every area of brain can cause late issues. Pediatric radiation oncologists try to avoid treating normal brain whenever possible, but especially the pituitary, hypothalamus, temporal lobes and/or the hippocampi, and optic apparatus. Work by Merchant has also shown that dosimetry impacts patient intelligence and quality of life in ways not otherwise predicted when examining low dose and intermediate dose region, in addition to standard high-dose regions.16-18 Some skull base histologies such as chordoma and chondrosarcoma are of special interest to proton therapy as well because required doses are often 70 to 79.2 Gy, and cannot be delivered with the same degree of OAR sparing with standard radiation therapy due to the proximity of the optic apparatus anteriorly, the brainstem posteriorly, and the hippocampi and cochleae laterally.19-22
Spine tumors
Spine tumors are rare for patients of all ages. The anatomical juxtaposition of the spine and normal structures that poorly tolerate high doses of radiation make protons a superior choice for spine radiation therapy in children. A single beam can typically address these lesions, and is best demonstrated in cases involving both kidneys, as a single posterior beam can spare the renal parenchyma and bowel.23,24
More than just the end of the beam, the edge of a proton beam can be given a sharper penumbra than a photon beam—a less-appreciated technique of proton therapy called edge blocking. Using brass apertures, this penumbra varies with depth and can be 2 mm from full dose to no dose laterally for shallow tumors. As such, clever use of proton beams can sculpt dose around the spinal cord via only posterior oblique fields, sparing most, if not all, of the kidney, bowel and cord. This can be of immense value when high doses of radiation are needed for chordomas or retreatment.25
Hodgkin lymphoma
When treating Hodgkin lymphoma, proton therapy is not typically the primary choice because doses in the pediatric setting are often < 30 Gy, and more typically 21 Gy, as directed in Children’s Oncology Group (COG) studies. Because these children have high cure rates, many live long enough to experience second malignancy and other late toxicities.26-28 In one series when radiation therapy was used definitively with much larger fields than today, upward of 40% of girls and women under 30 treated with radiation developed secondary breast cancer. The Childhood Cancer Study Group reported a high incidence of cardiac toxicity in this group as well. As a result, groups in Indiana and Florida have developed a method to avoid OARs in Hodgkins, including the female breast and heart. The newly opened COG protocol for Hodgkin disease allows for proton therapy based on these data.26, 29-32 Figure 2 shows a stage IIIA case with breast dose cut to about half photon dosing and intracranial sparing.
Tumors of the trunk (chest, abdomen, and pelvis)
Because proton beam therapy can avoid bowel, spleen, kidney, bladder, gonads, pancreas, and stomach exposure in ways otherwise difficult to address, pediatric tumors of the abdomen and pelvis can benefit from this treatment. When doses are relatively high, as with sarcomas and chordomas, proton use is relatively safe and well-tolerated even when doses exceed 60 Gy. When doses are both low and high, OAR sparing without very high integral dose remains a strength of protons. The use of protons in the pelvis and abdomen is not always superior to other modalities, however, and case-by-case comparison planning is often necessary. Tumors of the retroperitoneum can be addressed without significant dose to the bowel, which can be crucial in treating sarcomas.33,34 Figure 3 shows an Askin’s tumor with dose wrapped around the normal lung.
Retreatment
Retreatment is a new frontier in radiation oncology, and is rare in children. Despite this, several centers have retreated patients. At IUHPTC, we have treated a large number of patients a second time, many of whom were children. Data showing retreatment of gliomas and atypical teratoid/rhabdoid tumors (ATRTs) has recently been accepted for publication in two articles.35
We have also developed a novel method called “plugging” where beams are placed inside of other fields leaving dosimetric holes without dose, which is useful when retreating areas of the brain. This was used to deliver a second course of CSI without radiation-related toxicity for a patient treated with standard risk medulloblastoma, before the disease relapsed in a large spinal region lesion and outside of the CSI axis.25 More established advanced methods in proton therapy, such as “patch and through,” allow areas to receive no dose, while nearby areas see full dose.36-38 In “patch and through,” one beam stops (the patch) at the edge of another beam going all the way to the overall target volume’s end (the through). One can wrap dose around objects in the manner shown in Figure 3 using this method. Figure 4 shows a patient treated with a photon plan who experienced recurrence < 2 years later, and required full-dose retreatment. The plan also employed the “patch and through” technique around the optic apparatus.
Miscellaneous indications for pediatric proton therapy
Sarcoma of the extremity can be treated with multiple approaches. Brachytherapy serves as the primary means of addressing dose conformality and minimizing integral dose in sarcoma. In many instances, however, brachytherapy is not an option and sarcoma treatment will cross joint spaces and/or involve a large amount of the subcutaneous tissue, making lymphedema likely. In these cases, proton therapy can allow for joint sparing and lymphedema avoidance. These indications are dosimetric in nature and are unlikely to undergo randomized testing. Tumors of the hands, feet, wrist, and near the gonads may be optimal for protons, but a special physics evaluation is crucial given shallow depths and small fields in these areas. 39
Desmoid tumors of the trunk can often be treated to high dose using proton beam therapy without delivering dose to organs below the tumor given the nature of proton beam therapy. The lack of sharp margins makes field selection crucial, and the large fields of subcutaneous tissue are often impossible to treat with tangent fields due to field size and shape.40
Pediatric head and neck tumors are rare and commonly include nasopharyngioma and rhabdomyosarcoma. In this region, protons can treat the primary tumor to full dose while sparing OARs, and can treat the lymph nodes of the left and right necks without treating the esophagus, thus improving quality of life during, and potentially after, treatment.19,41,42
Contraindications for proton therapy
Wilm’s tumor classic fields
Because there is no intent to “spare” any tissue, use of protons for whole abdomen and flank therapy is not indicated. Protons cannot “spare” normal structures to a degree that would make sense. Additionally, the fields are shaped in such a way that protons could prevent proper dosimetry. For example, for the whole abdomen, gas content changes and overall shape and size could, in theory, make dose inaccu rate unless accounting for these variables, which would add complexity not found in a photon plan.43-45
Whole lung classic fields
The need to treat both lungs evenly with breathing motion and a beating heart makes photon anteroposterior and posteroanterior (AP-PA) fields the most logical approach. Dose is even with this method and motion is relatively well tolerated. Protons would not necessarily be able to handle the changes with the diaphragm well. Much like the Wilm’s cases, this classic large volume is so straightforward that protons do not add to the process, and could cause dosimetric issues as the lungs develop atelectasis or another change, altering the net “range.” Perhaps in the future we will see evidence that protons can spare the heart and whole lung. Until then, newer IMRT techniques may allow for timely treatment that proton therapy cannot address.46
Palliative care and rapid-start cases
While proton planning is inherently slower than photon planning, this difference will equalize as proton expertise increases. But for now, planning takes longer and cases requiring a quick start face delays due to processes intrinsic to protons. In addition, photons can be started in minutes; protons cannot. This reflects, in part, our comfort level with photons and electrons gained through vast opportunities for experience, which may change as experience with protons grows and processes are streamlined. In particular, the use of new methods that allow beams to be shaped without the standard requirement of customized field-shaping devices may decrease time from simulation to treatment. Because protons are slow to start, they are an unlikely contender for front-line emergency care. They can, however, be used with photons for rapid-onset situations such as a symptomatic leptomeningeal case, whereby one could start with photons and quickly shift to protons if indicated clinically.47,48 As long as protons cost more than photons, however, their use in pediatric palliative care will remain rare.
Rapidly changing anatomical areas
Unless gating is used, protons are more sensitive to movement than photons, since range often alters with movement. If a large gas bubble in the gut moves, for instance, dose could rise or fall as a patient’s shape changes. Or, as a patient breathes, a rib can shift its angle and become far thicker or far thinner. Without pre-emptive corrections, such changes can cause excessive dose irregularities, resulting in greater minimum dose. Patients who gain or lose weight rapidly throughout treatment are another example, as are patients with rapidly shrinking head and neck tumors, the latter of whom also require re-planning.49 A worst-case scenario is a child with a nasopharyngeal tumor that shrinks quickly, invalidating the proton plan and requiring rapid change and adaption. If not done, the protons could pass “through” the tumor area and could deliver unwanted dose to the temporal lobes — the very areas being avoided. If using protons in such cases where volumetric change could cause dose to be delivered to OARs, real-time imaging and adaptive planning are paramount. Without these tools, proton therapy is not likely the best option when anatomy is changing rapidly, even if the initial plan’s dosimetry is superior to other treatments. This point is crucial and underscores a weakness of proton beam therapy relating to protons’ unique capacity to stop quickly: increased sensitivity to change that can make a dosimetric miss far more likely.50,51 New planning software and proton spot scanning will allow more rapid response, but the process is difficult even with these tools.
Leukemic cranial fields
Brain radiation therapy is well handled by conventional techniques. However, avoiding the lids and lenses of young children is sometimes difficult with three-dimensional conformal radiotherapy (3DCRT), the standard of care for whole-brain therapy. In theory, IMRT could help this issue, but the shape of childrens’ brains, particularly in children under 5, can make even IMRT unable to spare the orbit while covering the frontal lobe and cribriform plate adequately. In these situations, protons are superior via oblique field use and distal blocking of the contralateral orbital region. While this is difficult to justify given cost issues, it will likely gain acceptance as proton therapy becomes more affordable. If costs were equal, the proton therapy approach would nearly always be at least equivalent, if not vastly superior, to the photon plan.52
Expanding indications for proton therapy
As noted, the primary reason proton therapy is not used for more pediatric indications such as whole brain is cost and time required for planning and treatment. Fortunately, proton therapy is more affordable than even a few years ago, and machinery prices will likely continue to drop. More centers are opening as well, making availability far greater than ever. Planning software is improving and spot scanning nozzles make construction of proton field-shaping devices a thing of the past. In time, these factors will likely result in the use of protons costing nearly the same as photons. While treatment ultimately may become financially bundled by disease, a disease will have a fixed pay ment regardless of treatment modality. As such, those who feel protons are superior will be allowed to use them as much as desired without penalty.53,54
Pediatric care and proton dosimetry
At the 16th International Society of Pediatric Neuro-Oncology (ISPNO) meeting in Singapore last June, several speakers presented pre-publication data regarding unexpected toxicity seen with proton therapy.55,56 These data, while not peer reviewed, were presented by leaders in the field from major institutions. One presentation examined 3 serious (NCI grade IV or higher) brainstem necrosis cases involving 3 proton centers.55 In each case, a child either died or suffered a significant impact.57,58
At IUPTC we had no such issues. Perhaps it was luck, or it may have been due to a method we developed to avoid dose uncertainty at the end of the Bragg peak.37 It is possible that beam modulation is needed to compensate for dose — an area not accounted for by planning systems. Our published method for avoiding toxicity is adaptable in a straightforward way to newer spot-scanning methods. The primary idea is the smearing of the distal beam end to avoid high dose areas caused by the Bragg peak’s higher biologic dose.37
Expanding research in proton therapy, like photon therapy, is underway across involved centers in plan robustness, image guidance, beam-selection algorithms, and biologic dose optimization. Each of these topics warrants a complete discussion that lies outside of the scope of this review.
Conclusions
Proton beam therapy for children and young adults is safe and can avoid large volumes of normal tissue due to unique characteristics of the beam’s distal edge. Several areas of pediatric cancer such as the CNS and the head and neck have relatively clear indications for proton therapy; less clear areas may benefit as well when proton cost is no longer an issue. Table 1 shows the current rough matrix of where things stand in 2015 for pediatric proton therapy. Clearly, excellent care can and has been achieved for years without proton therapy and will continue to be done when protons are not available. Equally clear, there are cases where protons are not superior even in children. As costs decrease and the capacity to react to changing target shape and range improves, it is likely that proton therapy will become more strongly indicated for some types of pediatric tumors.
Proton therapy is a complex treatment that demands extreme expertise and care since it is easier to miss targets when dose falloff is so abrupt. Unexpected toxicity is possible, but at least 1 published report outlines an accessible method that avoids these pitfalls.
Protons are not always better, even for small tumors near critical structures.59 But when contemplating which pediatric patients are best served by protons, physicians must consider several factors: the family’s ability to travel, the capacity of the proton center to treat children and, finally, the center’s expertise and support structures. Protons are an exciting aspect of pediatric radiation therapy that will ultimately become more available geographically as machine costs decrease. Proton therapy promises significant dosimetric improvements in many cases, but requires continued research and a sophisticated understanding of their limitations to use them well.
References
- Hug EB, Slater JD. Proton radiation therapy for pediatric malignancies: status report. Strahlenther Onkol. 1999;175(Suppl 2):89-91.
- Kirsch DG, Tarbell NJ. New technologies in radiation therapy for pediatric brain tumors: the rationale for proton radiation therapy. Pediatr Blood Cancer. 2004;42(5):461-464.
- Mailhot Vega RB, Kim J, Bussiere M, et al. Cost effectiveness of proton therapy compared with photon therapy in the management of pediatric medulloblastoma. Cancer. 2013;119(24):4299-4307.
- Yock, TI, Tarbell NJ. Technology insight: proton beam radiotherapy for treatment in pediatric brain tumors. Nat Clin Pract Onco. 2004;1(2):97-103. quiz 1 p following 111.
- Yock TI, Bhat S, Szymonifka J, et al. Quality of life outcomes in proton and photon treated pediatric brain tumor survivors. Radiother Oncol. 2014;113(1):89-94.
- Zhang R, Howell RB, Giebeler A, et al. A comparative study on the risks of radiogenic second cancers and cardiac mortality in a set of pediatric medulloblastoma patients treated with photon or proton craniospinal irradiation. Radiother Oncol. 2014;113(1):84-88.
- Zhang R, Howell RB, Giebeler A, et al. Comparison of risk of radiogenic second cancer following photon and proton craniospinal irradiation for a pediatric medulloblastoma patient. Phys Med Biol. 2013;58(4):807-823.
- Johnstone PA, McMullen KP, Buchsbaum JC, et al. Pediatric CSI: are protons the only ethical approach? Int J Radiat Oncol Biol Phys. 2013;87(2):228-230.
- Taddei PJ, Mirkovic D, Fontenot JD, et al. Reducing Stray Radiation Dose for a Pediatric Patient Receiving Proton Craniospinal Irradiation. Nucl Technol. 2009;168(1):108-112.
- Song S, Park HJ, Yoon JH, et al. Proton beam therapy reduces the incidence of acute haematological and gastrointestinal toxicities associated with craniospinal irradiation in pediatric brain tumors. Acta Oncol. 2014;53(9):1158-1164.
- Buchsbaum JC, Besemer A, Simmons J, et al. Supine proton beam craniospinal radiotherapy using a novel tabletop adapter. Med Dosim. 2013;38(1):70-76.
- Singhal M, Vincent A, Simoneaux V, et al. Overcoming the learning curve in supine pediatric proton craniospinal irradiation. J Am Coll Radiol. 2012;9(4):285-287.
- Athar BS, Bednarz B, Seco J, et al. Comparison of out-of-field photon doses in 6 MV IMRT and neutron doses in proton therapy for adult and pediatric patients. Phys Med Biol. 2010;55(10):2879-2891.
- Athar BS, Paganetti H. Comparison of second cancer risk due to out-of-field doses from 6-MV IMRT and proton therapy based on 6 pediatric patient treatment plans. Radiother Oncol. 2011;98(1):87-92.
- Taddei PJ, Mirkovic D, Fontenot JD, et al. Stray radiation dose and second cancer risk for a pediatric patient receiving craniospinal irradiation with proton beams. Phys Med Biol. 2009;54(8):2259-2275.
- Merchant TE, Kiehna EN, Li C, et al. Radiation dosimetry predicts IQ after conformal radiation therapy in pediatric patients with localized ependymoma. Int J Radiat Oncol Biol Phys. 2005;63(5):1546-1554.
- Merchant TE, Kiehna EN, Kun LE, et al. Phase II trial of conformal radiation therapy for pediatric patients with craniopharyngioma and correlation of surgical factors and radiation dosimetry with change in cognitive function. J Neurosurg. 2006;104(2 Suppl):94-102.
- Merchant TE, Conklin HM, Wu S, et al. Late effects of conformal radiation therapy for pediatric patients with low-grade glioma: prospective evaluation of cognitive, endocrine, and hearing deficits. J Clin Oncol. 2009;27(22):3691-3697.
- Rutz HP, Weber DC, Goitein G, et al. Postoperative spot-scanning proton radiation therapy for chordoma and chondrosarcoma in children and adolescents: initial experience at paul scherrer institute. Int J Radiat Oncol Biol Phys. 2008;71(1):220-225.
- Habrand JL, Schnieder R, Alapetite C, et al. Proton therapy in pediatric skull base and cervical canal low-grade bone malignancies. Int J Radiat Oncol Biol Phys. 2008;71(3):672-675.
- Hug EB, Muenter MW, Archambeau JO, et al. Proton radiotherapy in management of pediatric base of skull tumors. Int J Radiat Oncol Biol Phys. 2002;52(4):1017-1024.
- Catton C, O’Sullivan B, Bell R, et al. Chordoma: long-term follow-up after radical photon irradiation. Radioth Oncol. 1996;Oct;41(1):67-72.
- Miralbell R, Lomax A, Russo M. Potential role of proton therapy in the treatment of pediatric medulloblastoma/primitive neuro-ectodermal tumors: spinal theca irradiation. Int J Radiat Oncol Biol Phys.1997;38(4)805-811.
- Ray GL, Buchsbaum JC, McMullen MD. Definitive treatment of leptomeningeal spinal metastases in children. Pediatr Blood Cancer. 2013;60(11):1839-1841.
- McDonald MW, Wolanski MR, Simmons JW, et al. Technique for sparing previously irradiated critical normal structures in salvage proton craniospinal irradiation. Radiat Oncol. 2013;8:14.
- Lin HM, Teitell MA. Second malignancy after treatment of pediatric Hodgkin disease. J Pediatr Hematol Oncol. 2005;27(1):28-36.
- Hamilton VM, Norris C, Bunin N, et al. Cyclophosphamide-based, seven-drug hybrid and low-dose involved field radiation for the treatment of childhood and adolescent Hodgkin disease. J Pediatr Hematol Oncol. 2001;23(2):84-88.
- Hudson MM, Constine LS. Refining the role of radiation therapy in pediatric hodgkin lymphoma. Am Soc Clin Oncol Educ Book. 2012;616-620.
- Alehan D, Sahin M, Varan A, et al. Tissue Doppler evaluation of systolic and diastolic cardiac functions in long-term survivors of Hodgkin lymphoma. Pediatr Blood Cancer. 2012;58(2):250-255.
- Hoppe BS, Flampouri S, Lynch J, et al. Improving the therapeutic ratio in Hodgkin lymphoma through the use of proton therapy. Oncology. 2012;26(5):456-459,462-465.
- Hoppe BS, Flampouri S, Su Z, et al. Effective dose reduction to cardiac structures using protons compared with 3DCRT and IMRT in mediastinal Hodgkin lymphoma. Int J Radiat Oncol Biol Phys. 2012;84(2):449-455.
- Andolino DL Hoene T, Xiao L, et al. Dosimetric comparison of involved-field three-dimensional conformal photon radiotherapy and breast-sparing proton therapy for the treatment of Hodgkin’s lymphoma in female pediatric patients. Int J Radiat Oncol Biol Phys. 2011;81(4):e667-671.
- Lee CT, Bilton SD, Famiglietti RM, et al. Treatment planning with protons for pediatric retinoblastoma, medulloblastoma, and pelvic sarcoma: how do protons compare with other conformal techniques? Int J Radiat Oncol Biol Phys. 2005.63(2):362-372.
- Anderson PM, Pearson M. Novel therapeutic approaches in pediatric and young adult sarcomas. Curr Oncol Rep. 2006;8(4):310-315.
- Galle JO, McDonald MW, Simoneaux V, Buchsbaum JC. Reirradiation with proton therapy for recurrent gliomas. Int J Particle Therapy. 2015;2(1)11-19.
- Estabrook NC, McDonald MW, Hoene TA, et al. Proton radiotherapy for midline central nervous system lesions: A class solution. Oncology. 2015.
- Buchsbaum JC, McDonald MW, Johnstone PAS, et al. Range modulation in proton therapy planning: a simple method for mitigating effects of increased relative biological effectiveness at the end-of-range of clinical proton beams. Radiat Oncol. 2014;9:2.
- Calaminus G, Gobel U, Chrum J, et al. Proton beam therapy for loco-regional control of a recurrent mixed malignant germ cell tumor of the skull in a 22-month-old girl. Klin Padiatr. 2010;222(3):175-179.
- Ladra MM, Yock TI. Proton radiotherapy for pediatric sarcoma. Cancers. 2014;6(1):112-27.
- Kil WJ, Nichols RC, Kilkenny JW, et al. Proton therapy versus photon radiation therapy for the management of a recurrent desmoid tumor of the right flank: a case report. Radiat Oncol. 2012;7:178.
- Yamaoka M, Akiyama M, Yokokawa Y, et al. Multidisciplinary therapy including proton beam radiotherapy for a Ewing sarcoma family tumor of maxillary sinus in a 4-year-old girl. Head Neck. 2013;35(12):E386-390.
- Bolling, T, Weege J, Eich HT, et al. Acute and late side effects to salivary glands and oral mucosa after head and neck radiotherapy in children and adolescents. Results of the “Registry for the evaluation of side effects after radiotherapy in childhood and adolescence.” Head Neck. 2014.
- D’Angio GJ. The national wilms tumor study: a 40 year perspective. Lifetime Data Anal. 2007;13(4):463-470.
- Green DM. Jaffe N. Wilms’ tumor -- model of a curable pediatric malignant solid tumor. Cancer Treat Rev. 1978;5(3):143-172.
- Thomas, PR. Radiotherapy in Wilms’ tumour. Ann Acad Med Singapore. 1996;25(3):425-428.
- Yang JC, Wexler LH, Meyers PA, et al. Intensity-modulated radiation therapy with dose-painting for pediatric sarcomas with pulmonary metastases. Pediatr Blood Cancer. 2013; 60(10):1616-1620.
- Panoff J, Somoneaux RV, Shah N, et al. Radiation therapy at end of life in children. J Palliat Med. 2015;18(2):167-169.
- Rahn DA 3rd, Mundt AJ, Murphy JD, et al. Clinical outcomes of palliative radiation therapy for children. Pract Radiat Oncol. 2014.
- Mannina E Jr., Bartlett G, Wallance D, et al. Steroid-induced adaptive proton planning in a pediatric patient with low grade glioma: a case report and literature review. Pract Radiat Oncol. 2014;4(1):50-54.
- DeLaney, TF. Proton therapy in the clinic. Front Radiat Ther Oncol. 2011;43: 465-485.
- Beltran C, Roca M, Merchant TE. On the benefits and risks of proton therapy in pediatric craniopharyngioma. Int J Radiat Oncol Biol Phys. 2012;82(2):e281-287.
- Vincent A, Singhal M, Johnstone PAS, et al. Age-related changes in frontal lobe anatomy require alternatives to opposed lateral fields. Int J Particle Therapy. 2014;1(2):334-342.
- Mailhot Vega RB, Kim J, Hollander A, et al. Cost effectiveness of proton versus photon radiation therapy with respect to the risk of growth hormone deficiency in children. Cancer. 2015.
- Dvorak T, Wazer DE. Evaluation of potential proton therapy utilization in a market-based environment. J Am Coll Radiol. 2010;7(7):522-528.
- Wells E, Vezina G, Kilburn L, et al. Acute brain injury may be more severe from proton beam radiation (PBI) than from conventional radiation therapy (CRT) for young pediatric patients with posterior fossa tumors. Paper presented at: Singapore International Symposium on Pediatric Neuro-Oncology Meeting 2014, June 28-July 2, 2014.
- Buchsbaum, JC. Introduction to pediatric proton therapy. Paper presented at: Singapore International Symposium on Pediatric Neuro-Oncology Meeting 2014, June 28-July 2, 2014.
- Indelicato DJ, Flampouri S, Rotondo RL, et al. Incidence and dosimetric parameters of pediatric brainstem toxicity following proton therapy. Acta Onco. 2014;53(10):1298-1304.
- Freund D, Zhang R, Sanders M, et al. Predictive risk of radiation induced cerebral necrosis in pediatric brain cancer patients after VMAT versus proton therapy. Cancers. 2015;7(2):617-630.
- Conroy R, Gomes L, Owen C, et al. Clinical equipoise: protons and the child with craniopharyngioma. J Med Imaging Radiat Oncol. 2015;59(3):379-385.