Stereotactic Radiosurgery for Brain Metastases: Review of Existing Data and Future Directions
Images
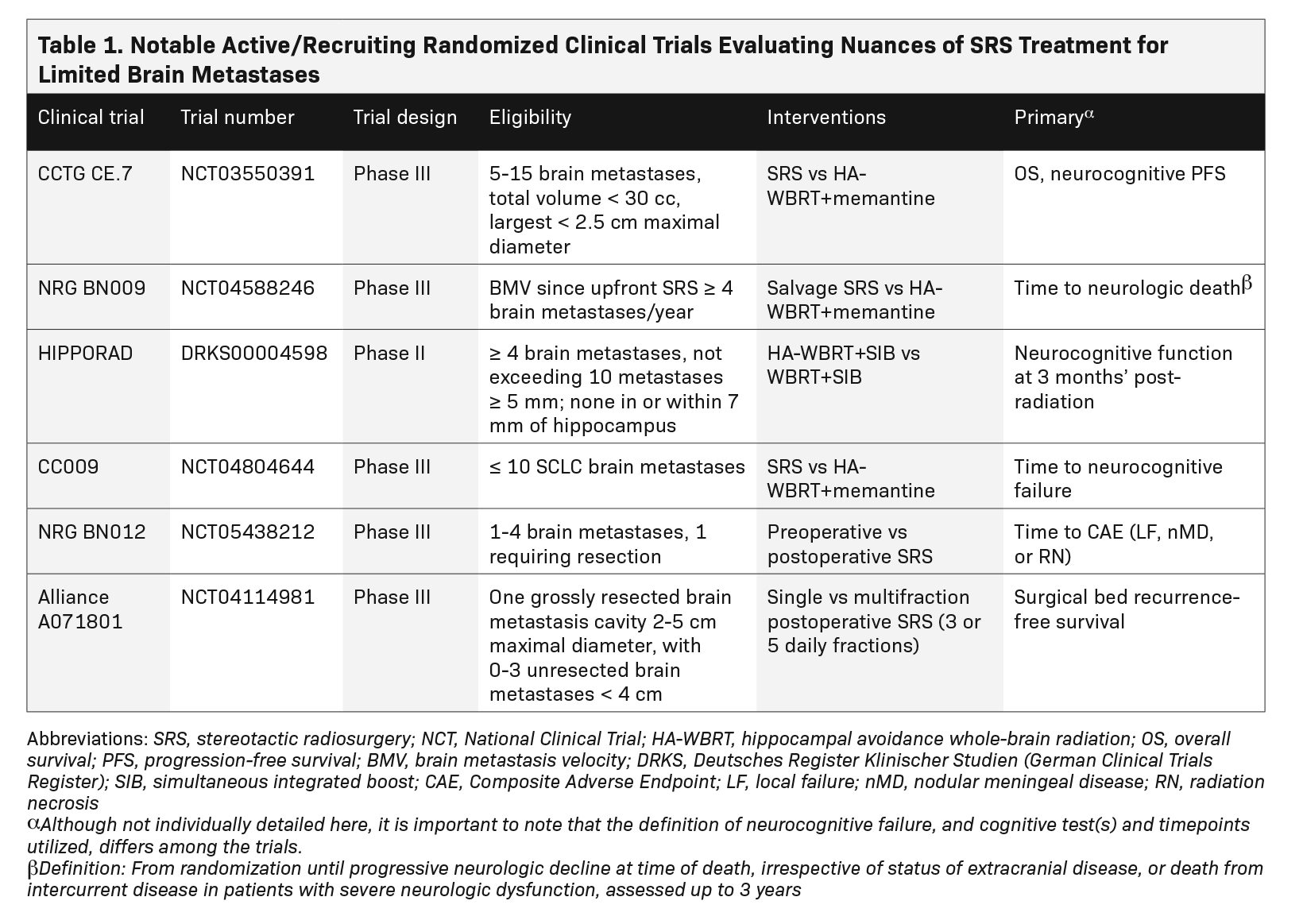
CME credits are available for this article here.
Abstract
Stereotactic radiosurgery (SRS) has become the standard of care for limited brain metastases to defer toxicities associated with whole-brain radiation therapy (WBRT). While WBRT decreases the appearance of new distant brain metastases, this is at the expense of worse cognitive decline without an overall survival benefit. However, the definition of limited brain metastases continues to be controversial and variably interpreted. Randomized trials are pending to evaluate whether contemporary WBRT techniques utilizing neuroprotective strategies such as hippocampal avoidance and memantine are more appropriate than SRS in specific clinical scenarios. The emerging use of SRS has also led to other discussions regarding postoperative cavity contouring, utility of preoperative SRS, and optimization of dose regimens, with growing support for fractionation of large tumors. In this narrative review, we will discuss the existing data and rationale supporting the predominance of SRS for brain metastases, and the evolving data for unanswered questions.
Keywords: radiosurgery, SRS, fractionated stereotactic radiation therapy, FSRT, radiation, radiation therapy, brain metastases, intracranial metastases
Survival of patients with metastatic cancer has markedly improved in recent years with development of better systemic therapies and surgical techniques, identification of targetable molecular mutations, and realization that aggressive treatment in patients with low volume (ie, oligometastatic) disease can be beneficial. With improved extracranial control and prognosis in many patients, optimizing intracranial control while minimizing late neurotoxicity has become paramount. Herein lies the appeal of stereotactic radiosurgery (SRS), which has supplanted whole-brain radiation therapy (WBRT) as the standard of care for limited brain metastases as supported by international guidelines1,2 and consistent with more recent systematic and narrative reviews.3-8 Practice patterns demonstrate a doubling of SRS use in the community from 2010 to 2015 with concomitant decline in WBRT.9 The numerical thresh- old of “limited” remains controversial but continues to be expanded with increasingly narrower indications for WBRT. SRS allows for dose escalation for increased local tumor control while sparing normal brain tissue to minimize toxicities, which can signifi- cantly impact quality of life. The growth of SRS has led to several clinical questions that are still being fleshed out, a few of which we discuss in this review: Is there a limit in the number/volume of metastases appropriate for SRS over WBRT techniques? What is the best sequencing of surgery and SRS? And, what is the optimal SRS dose/fractionation? Table 1 summarizes the active/recruiting trials aimed at elucidating some of these questions.
Methods
The PubMed database was searched using the terms brain metastases, cavity, and stereotactic radiosurgery, with article type selected for clinical trials, randomized controlled trials, meta-analyses, reviews, and systematic reviews.
SRS vs WBRT
We will first summarize the seminal data that support the routine use of SRS instead of WBRT, and the clinical scenarios when WBRT may be more appropriate. While WBRT decreases the appearance of new distant brain metastases (dBM), defined as development of new brain metastases different than the initial site,10 this is at the expense of worse cognitive decline without an overall survival benefit.11-18 WBRT originated in the 2D era when targeted irradiation of brain metastases was not possible and median survival of patients with metastases was only 2 to 4 months,19-21 such that treatment durability (up to ∼6 months with WBRT12) and late toxicity were not as relevant. Neurocognitive impairment (NCI) is markedly worse with conventional WBRT, especially in the domains of memory, learning, and executive function.13-15,17,18,22-25 Alliance N0574 trial reported 3 month NCI of 64% with SRS vs 92% with SRS plus WBRT, which persisted at 6 and 12 months, indicating it is not a reversible toxicity.25 Similarly, JCOG0504 reported a 2 times worse grade 2-4 NCI at 3 months post-WBRT vs salvage SRS (16 vs 8%, P = 0.048).14 Beyond NCI, other toxicities include fatigue, temporary alopecia, stroke, hearing loss, endocrinopathies, dry eye/mouth, and even retinopathy,26 all of which can impact quality of life.24,27 The 1-year rate of new brain metastases with SRS is approximately 50% (although dependent on systemic therapy), and WBRT bestows an absolute dBM reduction of approximately 20% to 30% (with most studies using 30 Gy in 10 fractions).11-14 A notable exception is melanoma, with no improvement in dBM, likely because of the radioresistant histology.18 Despite this general improvement in intracranial control, no data suggest an overall survival benefit (although no trial has been powered for overall survival); the rationale is that overall survival is driven by extracranial disease control, which is dictated by systemic therapy response.20 In addition, salvage SRS can often be done at the time of dBM without compromising outcomes (especially in patients with close follow-up and early salvage).24,28,29 Apart from overall survival, another endpoint of interest is cognitive decline, which can be secondary to treatment itself vs tumor progression. Several studies have used the somewhat ambiguous endpoint of neurologic death, essentially defined as progressive neurological decline at the time of death (regardless of systemic status).10 It is unknown whether WBRT improves the neurological death rate, as results from limited studies have been mixed,11,12,17,18,30 and it is difficult to define endpoint to begin with. However, several studies show that NCI is worse with WBRT rather than disease progression.13-15,23,24
The data above support the use of SRS instead of WBRT in patients with limited brain metastases and discourage the routine use of adjuvant WBRT after SRS. However, there is no universal consensus for defining limited. The initial SRS trials referenced above enrolled patients with less than 5 brain metastases. The large prospective observation study JLGK0901 then expanded that definition to up to 10, given no difference in overall survival when treated with SRS alone.31 Other studies followed in suit supporting greater than 10 brain metastases;32-34 however, given limitations in sample size/study design, controversy remains for greater than 10 and especially greater than 20 brain metastases.1,2 While high-level evidence for these specific clinical scenarios is lacking, many argue that one can extrapolate from the consistent conclusions in the aforementioned studies, and use salvage SRS to postpone WBRT toxicities for as long as possible. In their institutional experience of SRS for multiple brain metastases (range, 1-85 metastases; mean of 7; and median of 3), Yamamoto et al report that 85% of patients died of causes other than brain disease progression, regardless of brain metastasis number,35 again supporting the notion that overall survival is often not dictated by intracranial disease. Perhaps a more relevant proxy of intracranial tumor burden is not the number, but the volume of brain metastases.2,31,36 Again, the threshold above which overall survival favors a WBRT technique has not been established. One of the higher proposed cutoffs is 30 cc, used in the Canadian phase III CE.7 comparing SRS vs hippocampal avoidance (HA) WBRT plus memantine for 5 to 15 brain metastases (NCT03550391). Another potentially relevant parameter is brain metastasis velocity. Several studies have shown worse overall survival and neurocognitive death for first/second intracranial relapse in patients with high brain metastasis velocity.37,38 One proposed cutoff that may predict patients at high risk for neurologic death after SRS is greater than or equal to 4 metastases per year.37 This cutoff is being used in the phase III NRG Oncology BN009 study evaluating salvage HA-WBRT plus SRS vs SRS in patients with high brain metastasis velocity after upfront SRS (NCT04588246).
The above CE.7 and BN009 studies remind us that there are contemporary alternatives to the 2D-era WBRT, further complicating the discussion surrounding HA-WBRT with/without memantine with/without SRS boost. With neuroprotective strategies such as HA and memantine, and dose escalation with integrated SRS, WBRT may be more beneficial in a select subset of patients who may have poorer oncologic outcomes with repeated salvage SRS courses. HIPPORAD is an accruing phase II German trial evaluating HA-WBRT plus simultaneous integrated boost (SIB),39 with the hypothesis of improved tumor control with acceptable toxicity40 and less NCI than standard WBRT. The durability of WBRT 30 Gy is poor with 0% local control (LC) by 14 months for any size nonbreast adenocarcinoma and squamous cell carcinoma,41 in contrast to greater than 75% to 90% for SRS, even of large metastases greater than 2 cm.42-44 It should be noted that the aforementioned neuroprotective strategies help to reduce, but not eliminate, NCI after WBRT. In NRG CC001, even with the 26% relative risk reduction of NCI with the addition of HA to WBRT with memantine, the rates of neurocognitive toxicity remained over 50%, with a significant cognitive decline between 2 and 4 months following radiation.22 Similarly, even with the 22% relative risk reduction of NCI upon adding memantine to WBRT in RTOG 0614, the difference was not statistically significant (P = 0.59) given the likely insufficient statistical power from approximately 70% of patients dying by 6 months.45 And as with any drug, there are side effects that patients may not tolerate, such as fatigue, headache, nausea, body aches, and gastrointestinal disturbances.46
Even the topics of leptomeningeal disease (LMD) and certain histologies such as small cell lung cancer (SCLC), which are believed to have an increased rate of micrometastatic intracranial involvement, have garnered support for SRS in certain scenarios. Retrospective data support SRS for focal LMD to delay WBRT.47 While LMD is most commonly widely disseminated, if it is radiologically and symptom- atically focal, SRS may be done for potentially faster symptom relief and to postpone WBRT toxicity while minimizing time off systemic therapy (vs buying time to test the intracranial efficacy of a new systemic therapy). SCLC was excluded from the aforementioned seminal SRS trials given the traditional thought that disease is micrometastatic at onset. While SRS is controversial in patients with SCLC, there is growing evidence supporting its use with no overall survival detriment and dBM rates similar to non-SCLC, including FIRE-SCLC and a systematic review and meta-analysis of approximately 18,000 patients.48,49 CC009 is evaluating SRS vs HA-WBRT for less than or equal to 10 SCLC brain metastases (NCT04804644). Given the frequent use of WBRT in extensive stage SCLC, a move toward carefully selecting patients for SRS may save a large portion of patients from WBRT toxicities (due to adequate intracranial control with SRS or competing risk of non-neurologic death).
As mentioned above, the dBM failure rate with SRS is approximately 50% but dependent on systemic therapy. The development of more effective systemic therapies with intracranial efficacy, such as newer generation targeted therapies and immune checkpoint inhibitors (ICI) often used in non-small cell lung cancer (NSCLC), melanoma, and renal cell carcinoma, may subdue the high dBM rate. The decision to defer SRS for systemic therapy should be carefully considered, taking into account systemic therapy factors such as estimated time to response, response rate (partial vs complete), durability of response, and toxicity/compliance concerns, as well as patient/tumor factors such as symptoms, size, and distance from eloquent structures. Consider a patient with newly diagnosed EGFR-mutated NSCLC with innumerable punctate brain metastases and two 2-cm metastases, especially if symptomatic or near eloquent structures (but with sufficient distance to meet SRS constraints for organs at risk). It may be optimal to pursue upfront SRS to these 2 metastases with initiation of osimertinib, and a 6-week interval MRI (median time to response on osimertinib50) to re-evaluate the nonirradiated metastases. For melanoma brain metastases, the trial CheckMate 204 demonstrated that nivolumab/ ipilimumab can be an effective treatment with durable response in neurologically asymptomatic patients;51 however, median time to response is approximately 2 months, and efficacy is poor in neurologically symptomatic patients (22% response rate).51,52 Given the small sample size, it is unclear if this poor response is due to corticosteroid use vs disease burden/rapid progression, but it does highlight utility of local treatment (SRS vs resection) for large/symptomatic metastases.52 Other nuances of systemic therapy include sequencing and timing with SRS, with unclear interactions that may lead to synergy vs toxicity (radionecrosis).53 Promising studies suggest a potential overall survival benefit when SRS is administered concurrently with ICI (defined as within 4 weeks, given the long half-life of many ICIs) without increasing the risk of radionecrosis,54-56 although prospective data are needed.
In conclusion, SRS has become the standard of care for limited brain metastases, although that definition is variably interpreted and there may be select scenarios whereby WBRT techniques are more appropriate (currently under investigation). While awaiting those open trials, one should consider clinical factors such as prognosis/extracranial disease burden, systemic therapy options with anticipated intracranial efficacy, and intracranial tumor burden, with brain metastasis number, size, and volume likely all having relevance for clinical decision-making (ie, 100 punctate brain metastases may favor WBRT techniques vs starting a systemic therapy if anticipated to have good intracranial efficacy, while two 2-cm to 3-cm metastases would favor SRS given the limited number of metas- tases and desire for dose escalation given poor durable LC with WBRT41).
Perioperative SRS
For large brain metastases (not well-defined but typically > 2-4 cm), resection is often considered upfront, especially in patients with good performance status and limited/single brain metastases in surgically accessible locations. More important than an arbitrary size cutoff is the presence of symptoms from tumor/vasogenic edema, especially if not well-controlled with steroids or if systemic therapy initiation (ie, immunotherapy) is delayed by steroid use. As we will discuss below, tumor control with definitive SRS decreases with increasing tumor size, which historically has favored upfront resection. Even with a gross total resection (GTR), 1-year local failure rate is high at approximately 50% to 65%, and at least halved with adjuvant radiation.11,30,57 Patients who will start a systemic therapy with good intracranial efficacy (ie, targeted therapies like epidermal growth factor receptor [EGFR] inhibitors in NSCLC or dual immunotherapy in melanoma) arguably benefit less, and the decision for adjuvant radiation should be made on case-by-case basis. The Alliance N107c trial established cavity SRS as the standard of care over postoperative WBRT for the same reasons as discussed above.23,24 It should be noted that with contemporary studies, LC with cavity SRS is improved compared with historic rates referenced above (including N107c), now exceeding 90%.58 A variety of reasons could explain this observation: improved recognition of radionecrosis that may have historically been mistaken for local failure;59 learning curve of accurate target/cavity delineation, especially with improved MRI techniques; and use of higher equivalent dose/fractionated regimens (see SRS dose/fractionation section).
Regarding target delineation, cavity SRS contouring can vary considerably.60 Blood products and inflammation can make delineation of the cavity difficult.60 A decision must be made on whether to include a 1-2 mm cavity margin (essentially a less conformal SRS plan to account for uncertainty in cavity delineation),61 and whether to include the surgical corridor.60,62 While consensus contouring guidelines exist,60 these recommendations are based on expert opinion and not necessarily high-level evidence. Other nuances in cavity contouring include covering the preoperative extent of tumor contact with dura/falx/tentorium/venous sinus with or without additional margin.60 All these additional expansions may variably increase LC, but at the expense of increasing radionecrosis risk, and thus should be carefully considered. For example, it may be institutionally/individually decided to use a margin for small cavities less than 2 to 3 cm, especially if the cavity is not well-defined on imaging, and to include the surgical corridor if the cavity is superficially seated (ie, less than 1 cm) from the brain surface.
Another issue with the practical transition from WBRT to cavity SRS is the emergence of a new pattern of progression, which we will term nodular meningeal disease (nMD). This phenomenon is due to cerebrospinal fluid spread of tumor cells from surgery (which would have been irradiated with WBRT), and is likely underreported and mistaken for hematogeneous spread.63-65 Incidence of nMD after cavity SRS is approximately 10%,62,65 similar to the rates of classical LMD (cLMD). Unlike the “sugar coating” of cLMD, which is usually disseminated and associated with poor prognosis, nMD is typically focal nodules from iatrogenic spread.66 It most often presents on the pachymeninges (ie, dural-based nodules plus/minus a hypervascular tail akin to meningiomas64), although leptomeningeal nodules are also possible, and the two are not mutually exclusive. Given that nMD often behaves similarly to new parenchymal metastasis with no overall survival benefit of WBRT, SRS is reasonable treatment for when it occurs.66
These disadvantages of cavity SRS have fueled interest in preoperative SRS. This trend is similar to other disease sites such as rectal/esophageal cancer and soft-tissue sarcoma, whereby preoperative radiation is favored for simpler target delineation, smaller radiation field (for lower toxicity risk), and to avoid postoperative tumor hypoxia, which may contribute to radioresistance. In addition, preoperative SRS could potentially “sterilize” tumor cells to circumvent the nMD phenomenon. Preoperative SRS can be logistically difficult, especially for multifraction regimens, as typically resection is being considered for urgent decompression of large symptomatic metastases.
The multicenter cohort study Preoperative Radiosurgery for Brain Metastases (PROPS-B) is the largest cohort study to date (n = 242) evaluating outcomes of patients undergoing preoperative SRS for brain metastases. It demonstrated low rates of meningeal disease (~8% at 2 years, only one-third being cMD vs cLMD) and radionecrosis (∼7% at 2 years, half of which were symptomatic), with low postoperative surgical complications (4% of grade ≥ 3, similar to that expected for upfront resection).67 Only 2 patients (0.8%) had nonmetastatic brain lesions (primary brain tumors), which emphasizes the importance of pathologic proven metastatic disease in patients being considered for preoperative SRS. LC was reasonable for a single fraction with a median dose of 15 Gy (1-year local progression of 15%, and 2-year progression of 18%; median tumor size ∼10 cc, which correlates with ∼2.7 cm tumor diameter). SRS was delivered a median of 1 day before surgery (interquartile range of 1-3 days). Refer to the SRS dose/fractionation section below for further discussion of preoperative SRS dosing.
NRG BN012 is currently recruiting, comparing preoperative vs postoperative SRS in patients with 1-4 brain metastases, with 1 metastasis requiring resection (NCT05438212). With a calculated sample size of 224 patients, the primary hypothesis is that preoperative SRS will prolong time to a compositive adverse endpoint (CAE), defined as either local progression, radionecrosis, or nMD. Secondary endpoints include cognitive function and patient-reported outcomes. Doses are the same in both arms, 12-20 Gy in a single fraction; intact metastases are required to be less than 4 cm in diameter. Preoperative radiation is delivered within 7 days of surgery, while postoperative radiation is 10-30 days after surgery. Patients will be stratified by number of metastases (1 vs 2-4), breast cancer histology, cerebellar location, and whether targeted therapy/immunotherapy is used within 8 weeks of surgery or 4 weeks prior to registra- tion. Type of surgical resection (piecemeal vs en bloc) will also be evaluated given unclear association with nMD.68 Off trial, preoperative SRS may be pursued when logistically feasible and convenient for the patient, especially for colorectal cancer/breast histology or cerebellar location, which may have higher risk of nMD.69
SRS Dose/Fractionation
The most common late toxicity risk after SRS is radionecrosis, which typically occurs 3 months to a few years after SRS, and is symptomatic in approximately 33% to 50% of patients, requiring steroids or even resection.70 Factors associated with radionecrosis include increasing tumor size,71 increasing volume of normal brain irradiated (dictated by tumor size and gradient index),72 certain systemic therapies (eg, trastuzumab emtansine),73,74 and re-irradiation/repeat SRS.75 In practice, radiosurgery doses/regimens are dictated in part by Radiation Therapy Oncology Group (RTOG) 9005,71 with consideration for fractionation for large metastases (or metastases near critical structures like brainstem/optics) if logistically feasible. It is also influenced to a large degree by practitioner/institutional preference and certain patient factors (eg, prior radiation or future anticipated WBRT, and systemic therapy considerations). The most studied dosimetric parameters predictive of radionecrosis are V12 Gy or V14 Gy for single fraction (< 10-20 cc, perhaps < 30 cc for multiple targets).72,76 Some institutions consider fractionation if V12-14 Gy exceeds a certain threshold, but it is important to not underdose the tumor to meet an arbitrary V12-14 Gy volume (increasing the risk of local failure and thus repeat SRS, which can double/triple the risk of radionecrosis).72,75,77
SRS doses were first established by RTOG 9005, a phase I/II dose escalation study that sought to determine the maximum tolerated dose (MTD) for single-fraction radiosurgery of recurrent previously irradiated primary brain tumors or brain metastases.71 Notably, all participants had received conventionally fractionated radiation greater than or equal to 3 months from study entry (36% were primary brain tumors with prior median 60 Gy, and 64% were brain metastases with prior median 30 Gy), and tumors located in the brainstem or greater than 4.0 cm were excluded. Starting doses were inversely proportional to maximum tumor diameter in any plane (ie, 12 Gy for the largest tumor size stratum of 3.1-4.0 cm), and increased by 3 Gy increments if grade 3-5 CNS toxicity (i.e., severe neurologic symptoms from radiation necrosis or cerebral edema) at 3 months was less than 20%. Chronic CNS toxicity, meaning after 3 months, was also recorded to determine MTD. Treatment was permitted with either the Gamma Knife (Elekta) or a linear accelerator (linac), and dose was prescribed to the 50% to 90% isodose line to the enhancing tumor without a margin. The final recommended doses were 24 Gy for a maximum tumor diameter less than or equal to 2.0 cm (median tumor volume in this group was 3.6 cc, which corresponds to 1.8 cm diameter), 18 Gy for 2.1-3.0 cm (dropped from 21 Gy because of unacceptable chronic CNS toxicity), and 15 Gy for 3.1-4.0 cm.
RTOG 9005 was the first radiosurgery study by the RTOG group, and it is important to emphasize it was not establishing doses based on efficacy (ie, LC) but on toxicity, given that SRS was initially being considered in patients with limited treatment options who had undergone prior radiation. Hence, recommended radiation dose from 9005 inversely correlates with tumor size. In addition, MTD was not actually met in the less than or equal to 2.0 cm stratum as investigators were unwilling to escalate beyond 24 Gy (27 Gy would equate to 54 Gy Dmax on the Gamma Knife).71 In practice, how institutions dose the small metastases stratum varies significantly: Some follow 9005, quoting optimal balance of LC and radionecrosis,42 others do not exceed 20-21 Gy,42,78 while others further stratify the less than or equal to 2.0 cm group (ie, 24 Gy for < 1.0 cm, and 22 Gy for 1.0-2.0 cm, as is done on protocol in BN012). Of interest, 9005 reported that linac treatment was associated with higher local recurrence compared with the Gamma Knife, so initially it was thought that higher heterogeneity (the internal “hot spots” inherent to Gamma Knife planning given dose is prescribed to the 50% isodose line) yielded better LC. However, this observation was not seen in the subsequent RTOG 950879 or comparative studies, including a single-institutional randomized trial.80 It may be that minimum dose (Dmin; akin to spinal metastases) and not maximum dose (Dmax) is more important for LC.81,82 Regarding technique, a recently published international guideline thoughtfully discusses nuances regarding treatment planning of small brain metastases.83 While planning margins were not used in 9005, clinical decision was based on the treatment platform and institutional/physician preference. For example, Gamma Knife, CyberKnife (Accuray Inc.), and the specialist linac Novalis (Varian) have submillimeter positional accuracy, yet for nonframe-based SRS some centers use no margin while others round up to 1 mm (seemingly innocuous, but it can double the ir- radiated volume and potentially increase the radionecrosis risk).76,83 Differences in contouring due to partial volume effect on MRI can also unintentionally add/omit margin.83
Unlike the small metastases stratum, multiple series have shown that large metastases (> 2-2.5 cm) have poor LC with 9005 single-fraction dosing (1 year LC ~40%-50%).43,78,84,85 Alluding to the principles of radiobiology, fractionated stereotactic radiation therapy (FSRT) has been employed to improve LC/radionecrosis risk. While randomized data do not yet exist, it is supported by comparative data and meta-analyses for intact and resected metastases.86,87 With development of noninvasive frameless methods, hypofractionation can also be applied to the Gamma Knife.88 Several comparative studies from Minniti et al support FSRT for large intact and resected metastases with 1-year LC improved to greater than 90% (even with a median intact metastases diameter of ~3.2 cm44 and median cavity ~3.9 cm,89 although intact melanoma may still have suboptimal LC),44,89 and overall radionecrosis approximately halved to less than or equal to 10%.44,89,90 The ideal multifraction regimen is not well established, although there is growing evidence for 27 Gy in 3 consecutive fractions (with better LC rates than 24 Gy, and less radionecrosis than 30 Gy)44,89-92 and 30-35 Gy in 5 consecutive frac- tions.8,93 There is an ongoing Italian randomized study evaluating 27 Gy in 3 fractions vs 35 Gy in 5 fractions for intact metastases.94
In addition, Alliance A071801 is currently comparing single-fraction SRS vs hypofractionated radiosurgery for resection cavities (NCT04114981), with fractionation of 27 Gy in 3 fractions for cavities less than 30 cc, or approximately 3.9-cm diameter, and 30 Gy in 5 fractions for greater than 30 cc. While awaiting these results, in practice some institutions utilize a volumetric cutoff to decide on 3 vs 5 fractions, similar to the Alliance A071801 study. Two separate institutional phase I/II dose studies reported MTD to be 27 Gy in 3 fractions (all cavities, MTD determined individually for 2-3 cm and 3-4 cm diameter cavities)28,92 and 32.5 Gy in 5 fractions (20 of 25 were cavities; 3-6 cm diameter allowed, median 3.3-cm diameter).95 Of note, these MTD and other dose finding studies often focus exclusively on cavity or intact metastases, and one must be cautious extrapolating for the other scenario. Logically it would seem that the risk of radionecrosis is higher for a similarly sized cavity given surgical manipulation and a larger volume irradiated after including additional margins (which can potentially double the volume irradiated). Emory’s phase I MTD 5-fraction study, for example, preliminarily reported toxicities exclusively in the cavity group.95 In a similar vein, Minniti et al compared intact vs cavity FSRT with 27 Gy in 3 fractions, and reported similarly high 1-year LC (> 90%), although radionecrosis and meningeal disease were lower for the intact metastases (which also supports definitive SRS in cases whereby surgery is not required for rapid relief of neurological deficits).90 Thus, in practice 30 Gy in 3 fractions or 35 Gy in 5 fractions are reasonable to consider (accepting potentially higher radionecrosis risk to optimize local control),77 especially for intact metastases with radioresistant histologies such as colorectal cancer or possibly melanoma.44
It may even be that FSRT is beneficial for small intact or resected metastases less than or equal to 2.0 cm given radiobiologic properties. In the meantime, a large meta-analysis supports FSRT for cavities87 (although most cavities are large and do not shrink significantly to be < 2.0 cm for it to be a relevant issue). The Alliance A071801 and BN012 studies may be useful in answering this question for cavities.
Given the likely higher necrosis risks with cavity radiation, we return to the topic of preoperative SRS to discuss dosing. Initial studies had dose reduced from RTOG 9005 by approximately 10% to 20% and found unacceptably higher local failures;67 this dose reduction attempt was likely driven by the fact that the vast majority of resections are GTR and postoperative hypoxia is not present. The PROPS-BM multicenter cohort study used a median 15-Gy SRS dose (SRS dose/fractionation was left to institutional protocol, although 99% were treated with a single fraction) and reported a low radionecrosis rate of 7% with median tumor size approximately 10 cc (which correlates with an approximate 2.7-cm tumor diameter). Subtotal resection, although infrequent (6%), was associated with worse local recurrence, which supports optimizing rather than reducing SRS dose. Notably, BN012 is a single-fraction study (as N107c utilized single fraction, and it was the landmark study to establish cavity SRS as the standard of care over WBRT) and does not dose reduce for the preoperative arm. Off trial, preoperative FSRT instead of SRS may alternatively be pursued for certain large metastases (eg, a 3-cm single colorectal cancer metastasis), assuming logistics and the urgency of surgery permits it (typically radiation is done 24-48 hours before resection).
Conclusion
While the increasing use of brain SRS in the last decade is logical given the accumulating high-level evidence reasserting its advantages over WBRT, many questions and uncertainties remain with regard to its application. The point at which patient-centric outcomes tip in favor of contemporary WBRT techniques over SRS is not clear, although several trials are evaluating promising metrics such as metastases volume and velocity. For large metastases, due to the variability of cavity contouring and identification of a new pattern of iatrogenic meningeal progression, preoperative SRS is currently under evaluation. In addition, large metastases not requiring surgical decompression are increasingly being treated by multifractionated regimens for potentially improved control and radionecrosis rates, although the optimal regimen is not clear. While awaiting answers from clinical trials, practice is influenced in part by institutional/individual preferences and interpretation/extrapolation of existing data.
References
- Gondi V, Bauman G, Bradfield L, et al. Radiation Therapy for Brain Metastases: An ASTRO Clinical Practice Guideline. Pract Radiat Oncol. 2022;12(4):265-282. doi:10.1016/j.prro.2022.02.003
- Milano MT, Chiang VLS, Soltys SG, et al. Executive summary from American Radium Society’s appropriate use criteria on neurocognition after stereotactic radiosurgery for multiple brain metastases. Neuro Oncol. Dec 18 2020;22(12):1728-1741. doi:10.1093/neuonc/noaa192
- Niranjan A, Monaco E, Flickinger J, Lunsford LD. Guidelines for multiple brain metastases radiosurgery. Prog Neurol Surg. 2019;34:100-109. doi:10.1159/000493055
- Soliman H, Das S, Larson DA, Sahgal A. Stereotactic radiosurgery (SRS) in the modern management of patients with brain metastases. Oncotarget. 2016;7(11):12318-12330. doi:10.18632/oncotarget.7131
- Grenzelia M, Zygogianni A, Grapsa D, et al. Limited cerebral metastases in NSCLC: a literature review of SRS versus whole-brain radiotherapy. Cancer Diagn Progn. 2022;2(6):609-619. doi:10.21873/cdp.10150
- Chaung KV, Sloan AE, Choi S. Limited brain metastases: a narrative review. Ann Palliat Med. 2021;10(5):6016-6027. doi:10.21037/apm-21-363
- edmond KJ, De Salles AAF, Fariselli L, et al. Stereotactic radiosurgery for postoperative metastatic surgical cavities: a critical review and International Stereotactic Radiosurgery Society (ISRS) practice guidelines. Int J Radiat Oncol Biol Phys. 2021;111(1):68-80. doi:10.1016/j.ijrobp.2021.04.016
- Minniti G, Niyazi M, Andratschke N, et al. Current status and recent advances in resection cavity irradiation of brain metastases. Radiat Oncol. 2021;16(1):73. doi:10.1186/s13014-021-01802-9
- Barbour AB, Jacobs CD, Williamson H, et al. Radiation therapy practice patterns for brain metastases in the United States in the stereotactic radiosurgery era. Adv Radiat Oncol. 2020;5(1):43-52. doi:10.1016/j.adro.2019.07.012
- Patchell RA, Tibbs PA, Walsh JW, et al. A randomized trial of surgery in the treatment of single metastases to the brain. N Engl J Med. 1990;322(8):494-500. doi:10.1056/NEJM199002223220802
- Kocher M, Soffietti R, Abacioglu U, et al. Adjuvant whole-brain radiotherapy versus observation after radiosurgery or surgical resection of one to three cerebral metastases: results of the EORTC 22952-26001 study. J Clin Oncol. 2011;29(2):134-141. doi:10.1200/JCO.2010.30.1655
- Aoyama H, Shirato H, Tago M, et al. Stereotactic radiosurgery plus whole-brain radiation therapy vs stereotactic radiosurgery alone for treatment of brain metastases: a randomized controlled trial. JAMA. 2006;295(21):2483-91. doi:10.1001/jama.295.21.2483
- Churilla TM, Ballman KV, Brown PD, et al. Stereotactic radiosurgery with or without whole-brain radiation therapy for limited brain metastases: a secondary analysis of the North Central Cancer Treatment Group N0574 (Alliance) randomized controlled trial. Int J Radiat Oncol Biol Phys. 2017;99(5):1173-1178. doi:10.1016/j.ijrobp.2017.07.045
- Chang EL, Wefel JS, Hess KR, et al. Neurocognition in patients with brain metastases treated with radiosurgery or radiosurgery plus whole-brain irradiation: a randomised controlled trial. Lancet Oncol. 2009;10(11):1037-1044. doi:10.1016/S1470-2045(09)70263-3
- Sun A, Bae K, Gore EM, et al. Phase III trial of prophylactic cranial irradiation compared with observation in patients with locally advanced non-small-cell lung cancer: neurocognitive and quality-of-life analysis. J Clin Oncol. 2011;29(3):279-286. doi:10.1200/JCO.2010.29.6053
- Gondi V, Paulus R, Bruner DW, et al. Decline in tested and self-reported cognitive functioning after prophylactic cranial irradiation for lung cancer: pooled secondary analysis of Radiation Therapy Oncology Group randomized trials 0212 and 0214. Int J Radiat Oncol Biol Phys. 2013;86(4):656-664. doi:10.1016/j. ijrobp.2013.02.033
- Kayama T, Sato S, Sakurada K, et al. Effects of surgery with salvage stereotactic radiosurgery versus surgery with whole-brain radiation therapy in patients with one to four brain metastases (JCOG0504): A phase III, noninferiority, randomized controlled trial. J Clin Oncol. 2018;36(33):3282-3289. doi:10.1200/ JCO.2018.78.6186
- Hong AM, Fogarty GB, Dolven-Jacobsen K, et al. Adjuvant whole-brain radiation therapy compared with observation after local treatment of melanoma brain metastases: a multicenter, randomized phase III trial. J Clin Oncol. 2019;37(33):3132-3141. doi:10.1200/JCO.19.01414
- Gaspar L, Scott C, Rotman M, et al. Recursive partitioning analysis (RPA) of prognostic factors in three Radiation Therapy Oncology Group (RTOG) brain metastases trials. Int J Radiat Oncol Biol Phys. 1997;37(4):745-751. doi:10.1016/s0360-3016(96)00619-0
- Sperduto PW, Mesko S, Li J, et al. Beyond an updated graded prognostic assessment (Breast GPA): a prognostic index and trends in treatment and survival in breast cancer brain metastases from 1985 to today. Int J Radiat Oncol Biol Phys. 2020;107(2):334-343. doi:10.1016/j.ijrobp.2020.01.051
- Sperduto PW, Kased N, Roberge D, et al. Summary report on the graded prognostic assessment: an accurate and facile diagnosis-specific tool to estimate survival for patients with brain metastases. J Clin Oncol. 2012;30(4):419-425. doi:10.1200/JCO.2011.38.0527
- Brown PD, Gondi V, Pugh S, et al. Hippocampal avoidance during whole-brain radiotherapy plus memantine for patients with brain metastases: phase III trial NRG Oncology CC001. J Clin Oncol. 2020;38(10):1019-1029. doi:10.1200/JCO.19.02767
- Brown PD, Ballman KV, Cerhan JH, et al. Postoperative stereotactic radiosurgery compared with whole brain radiotherapy for resected metastatic brain dis- ease (NCCTG N107C/CEC·3): a multicentre, randomised, controlled, phase 3 trial. Lancet Oncol. 2017;18(8):1049-1060. doi:10.1016/S1470-2045(17)30441-2
- Palmer JD, Klamer BG, Ballman KV, et al. Association of long-term outcomes with stereotactic radiosurgery vs whole-brain radiotherapy for resected brain metastasis: a secondary analysis of the N107C/CEC.3 (Alliance for Clinical Trials in Oncology/Canadian Cancer Trials Group) randomized clinical trial. JAMA Oncol. 2022;8(12):1809-1815. doi:10.1001/jamaoncol.2022.5049
- Brown PD, Jaeckle K, Ballman KV, et al. Effect of radiosurgery alone vs radiosurgery with whole brain radiation therapy on cognitive function in patients with 1 to 3 brain metastases: a randomized clinical trial. JAMA. 2016;316(4):401-409. doi:10.1001/jama.2016.9839
- Chan L, Sneed PK, Horton JC. Damage to the superior retinae after 30 Gy whole-brain radiation. Adv Radiat Oncol. 2021;6(4):100706. doi:10.1016/j. adro.2021.100706
- Soffietti R, Kocher M, Abacioglu UM, et al. A European organisation for research and treatment of cancer phase III trial of adjuvant whole-brain radiotherapy versus observation in patients with one to three brain metastases from solid tumors after surgical resection or radiosurgery: quality-of-life results. J Clin Oncol. 2013;31(1):65-72. doi:10.1200/JCO.2011.41.0639
- Rahimy E, Dudley SA, von Eyben R, et al. Phase I/II dose-escalation trial of 3-fraction stereotactic radiosurgery for resection cavities from large brain metas- tases: health-related quality of life outcomes. Am J Clin Oncol. 2021;44(11):588-595. doi:10.1097/COC.0000000000000868
- Koiso T, Yamamoto M, Kawabe T, et al. Follow-up results of brain metastasis patients undergoing repeat Gamma Knife radiosurgery. J Neurosurg. 2016;125(Suppl 1):2-10. doi:10.3171/2016.6.GKS161404
- Patchell RA, Tibbs PA, Regine WF, et al. Postoperative radiotherapy in the treatment of single metastases to the brain: a randomized trial. JAMA. 1998;280(17):1485-1489.
- Yamamoto M, Serizawa T, Shuto T, et al. Stereotactic radiosurgery for patients with multiple brain metastases (JLGK0901): a multi-institutional prospective observational study. Lancet Oncol. 2014;15(4):387-95. doi:10.1016/S1470-2045(14)70061-0
- Li J, Ludmir E, Guha-Thakurta N, al e. Stereotactic radiosurgery versus whole-brain radiation therapy for patients with 4-15 brain metastases: a phase III randomized controlled trial. [Abstract]. American Society for Radiation Oncology 2020 Annual Virtual Meeting.
- Hughes RT, Masters AH, McTyre ER, et al. Initial SRS for patients with 5 to 15 brain metastases: results of a multi-institutional experience. Int J Radiat Oncol Biol Phys. 2019;104(5):1091-1098. doi:10.1016/j.ijrobp.2019.03.052
- Yamamoto M, Higuchi Y, Sato Y, Aiyama H, Kasuya H, Barfod BE. Stereotactic radiosurgery for patients with 10 or more brain metastases. Prog Neurol Surg. 2019;34:110-124. doi:10.1159/000493056
- Yamamoto M, Kawabe T, Barfod BE. How many metastases can be treated with radiosurgery? Prog Neurol Surg. 2012;25:261-72. doi:10.1159/000331199
- Likhacheva A, Pinnix CC, Parikh NR, et al. Predictors of survival in contemporary practice after initial radiosurgery for brain metastases. Int J Radiat Oncol Biol Phys. 2013;85(3):656-661. doi:10.1016/j.ijrobp.2012.05.047
- LeCompte MC, Hughes RT, Farris M, et al. Impact of brain metastasis velocity on neurologic death for brain metastasis patients experiencing distant brain failure after initial stereotactic radiosurgery. J Neurooncol. 2020;146(2):285-292. doi:10.1007/s11060-019-03368-9
- McTyre ER, Soike MH, Farris M, et al. Multi-institutional validation of brain metastasis velocity, a recently defined predictor of outcomes following stereotac- tic radiosurgery. Radiother Oncol. 2020;142:168-174. doi:10.1016/j.radonc.2019.08.011
- Grosu AL, Frings L, Bentsalo I, et al. Whole-brain irradiation with hippocampal sparing and dose escalation on metastases: neurocognitive testing and biological imaging (HIPPORAD) - a phase II prospective randomized multicenter trial (NOA-14, ARO 2015-3, DKTK-ROG). BMC Cancer. 2020;20(1):532. doi:10.1186/ s12885-020-07011-z
- Westover KD, Mendel JT, Dan T, et al. Phase II trial of hippocampal-sparing whole brain irradiation with simultaneous integrated boost for metastatic cancer. >Neuro Oncol. 2020;22(12):1831-1839. doi:10.1093/neuonc/noaa092
- Nieder C, Berberich W, Schnabel K. Tumor-related prognostic factors for remission of brain metastases after radiotherapy. Int J Radiat Oncol Biol Phys. 1997;39(1):25-30. doi:10.1016/s0360-3016(97)00154-5
- Mohammadi AM, Schroeder JL, Angelov L, et al. Impact of the radiosurgery prescription dose on the local control of small (2 cm or smaller) brain metastases. J Neurosurg. 2017;126(3):735-743. doi:10.3171/2016.3.JNS153014
- Vogelbaum MA, Angelov L, Lee SY, Li L, Barnett GH, Suh JH. Local control of brain metastases by stereotactic radiosurgery in relation to dose to the tumor margin. J Neurosurg. 2006;104(6):907-912. doi:10.3171/jns.2006.104.6.907
- Minniti G, Scaringi C, Paolini S, et al. Single-fraction versus multifraction (3 × 9 Gy) stereotactic radiosurgery for large (> 2 cm) brain metastases: a compara- tive analysis of local control and risk of radiation-induced brain necrosis. Int J Radiat Oncol Biol Phys. 2016;95(4):1142-1148. doi:10.1016/j.ijrobp.2016.03.013
- Brown PD, Pugh S, Laack NN, et al. Memantine for the prevention of cognitive dysfunction in patients receiving whole-brain radiotherapy: a randomized, double-blind, placebo-controlled trial. Neuro Oncol. 2013;15(10):1429-1437. doi:10.1093/neuonc/not114
- Scampoli C, Cammelli S, Galietta E, et al. Memantine in the prevention of radiation-induced brain damage: a narrative review. Cancers (Basel). 2022;14(11):2736. doi:10.3390/cancers14112736
- Wolf A, Donahue B, Silverman JS, Chachoua A, Lee JK, Kondziolka D. Stereotactic radiosurgery for focal leptomeningeal disease in patients with brain metas- tases. J Neurooncol. 2017;134(1):139-143. doi:10.1007/s11060-017-2497-6
- Gaebe K, Li AY, Park A, et al. Stereotactic radiosurgery versus whole brain radiotherapy in patients with intracranial metastatic disease and small-cell lung cancer: a systematic review and meta-analysis. Lancet Oncol. 2022;23(7):931-939. doi:10.1016/S1470-2045(22)00271-6
- Rusthoven CG, Yamamoto M, Bernhardt D, et al. Evaluation of first-line radiosurgery vs whole-brain radiotherapy for small cell lung cancer brain metastases: the FIRE-SCLC cohort study. JAMA Oncol. 2020;6(7):1028-1037. doi:10.1001/jamaoncol.2020.1271
- Reungwetwattana T, Nakagawa K, Cho BC, et al. CNS response to osimertinib versus standard epidermal growth factor receptor tyrosine kinase inhibitors in patients with untreated EGFR-mutated advanced non-small-cell lung cancer. J Clin Oncol. 2018:JCO2018783118. doi:10.1200/JCO.2018.78.3118
- Tawbi HA, Forsyth PA, Algazi A, et al. Combined nivolumab and ipilimumab in melanoma metastatic to the brain. N Engl J Med. 2018;379(8):722-730. doi:10.1056/NEJMoa1805453
- Tawbi HA, Forsyth PA, Hodi FS, et al. Safety and efficacy of the combination of nivolumab plus ipilimumab in patients with melanoma and asymptomatic or symptomatic brain metastases (CheckMate 204). Neuro Oncol. 2021;23(11):1961-1973. doi:10.1093/neuonc/noab094
- Dohm AE, Tang JD, Mills MN, et al. Clinical outcomes of non-small cell lung cancer brain metastases treated with stereotactic radiosurgery and immune checkpoint inhibitors, EGFR tyrosine kinase inhibitors, chemotherapy and immune checkpoint inhibitors, or chemotherapy alone. J Neurosurg. 2022:1-8. doi:10.3171/2022.9.JNS221896
- Lehrer EJ, Kowalchuk RO, Gurewitz J, et al. Concurrent administration of immune checkpoint inhibitors and single fraction stereotactic radiosurgery in patients with non-small cell lung cancer, melanoma, and renal cell carcinoma brain metastases is not associated with an increased risk of radiation necro- sis over nonconcurrent treatment: an international multicenter study of 657 patients. Int J Radiat Oncol Biol Phys. 2023; S0360-3016(23)00057-3. doi:10.1016/j. ijrobp.2023.01.017
- Qian JM, Yu JB, Kluger HM, Chiang VL. Timing and type of immune checkpoint therapy affect the early radiographic response of melanoma brain metastases to stereotactic radiosurgery. Cancer. 2016;122(19):3051-3058. doi:10.1002/cncr.30138
- Lehrer E, Gurewitz J, Malouff T, et al. Concurrent vs. sequential stereotactic radiosurgery and immune checkpoint inhibition in melanoma brain metastases: an International Cooperative Group study. [Abstract]. American Society for Radiation Oncology 2021 Annual Meeting.
- Mahajan A, Ahmed S, McAleer MF, et al. Post-operative stereotactic radiosurgery versus observation for completely resected brain metastases: a single-centre, randomised, controlled, phase 3 trial. Lancet Oncol. 2017;18(8):1040-1048. doi:10.1016/S1470-2045(17)30414-X
- Shi S, Sandhu N, Jin MC, et al. Stereotactic radiosurgery for resected brain metastases: single-institutional experience of over 500 cavities. Int J Radiat Oncol Biol Phys. 2020;106(4):764-771. doi:10.1016/j.ijrobp.2019.11.022
- Breen W, Dooley K, Twohy E, et al. Patterns of failure after stereotactic radiosurgery vs. whole brain radiotherapy for resected brain metastases: central imaging review of the N107C/CEC.3 (Alliance) phase III clinical trial. [Abstract]. American Society for Radiation Oncology 2022 Annual Virtual Meeting.
- Soliman H, Ruschin M, Angelov L, et al. Consensus contouring guidelines for postoperative completely resected cavity stereotactic radiosurgery for brain metastases. Int J Radiat Oncol Biol Phys. 2018;100(2):436-442. doi:10.1016/j.ijrobp.2017.09.047
- Soltys SG, Adler JR, Lipani JD, et al. Stereotactic radiosurgery of the postoperative resection cavity for brain metastases. Int J Radiat Oncol Biol Phys. 2008;70(1):187-93. doi:10.1016/j.ijrobp.2007.06.068
- Shi S, Sandhu N, Jin M, et al. Stereotactic radiosurgery for resected brain metastases: does the surgical corridor need to be targeted? Pract Radiat Oncol. 2020;10(5):e363-e371. doi:10.1016/j.prro.2020.04.009
- Brennan C, Yang TJ, Hilden P, et al. A phase 2 trial of stereotactic radiosurgery boost after surgical resection for brain metastases. Int J Radiat Oncol Biol Phys. 2014;88(1):130-6. doi:10.1016/j.ijrobp.2013.09.051
- Turner BE, Prabhu RS, Burri SH, et al. Nodular leptomeningeal disease—a distinct pattern of recurrence after postresection stereotactic radiosurgery for brain metastases: a multi-institutional study of interobserver reliability. Int J Radiat Oncol Biol Phys. 2020;106(3):579-586. doi:10.1016/j.ijrobp.2019.10.002
- Cagney DN, Lamba N, Sinha S, et al. Association of neurosurgical resection with development of pachymeningeal seeding in patients with brain metastases.JAMA Oncol. 2019;5(5):703-709. doi:10.1001/jamaoncol.2018.7204
- Prabhu RS, Turner BE, Asher AL, et al. A multi-institutional analysis of presentation and outcomes for leptomeningeal disease recurrence after surgical resec- tion and radiosurgery for brain metastases. Neuro Oncol. 2019;21(8):1049-1059. doi:10.1093/neuonc/noz049
- Prabhu RS, Dhakal R, Vaslow ZK, et al. Preoperative radiosurgery for resected brain metastases: the PROPS-BM multicenter cohort study. Int J Radiat Oncol Biol Phys. 2021;111(3):764-772. doi:10.1016/j.ijrobp.2021.05.124
- Vogelbaum MA, Yu HM. Nodular leptomeningeal disease after surgery for a brain metastasis-should we be concerned? Neuro Oncol. 2019;21(8):959-960. doi:10.1093/neuonc/noz081
- Atalar B, Modlin LA, Choi CY, et al. Risk of leptomeningeal disease in patients treated with stereotactic radiosurgery targeting the postoperative resection cav- ity for brain metastases. Int J Radiat Oncol Biol Phys. 2013;87(4):713-8. doi:10.1016/j.ijrobp.2013.07.034
- Walker AJ, Ruzevick J, Malayeri AA, et al. Postradiation imaging changes in the CNS: how can we differentiate between treatment effect and disease progres- sion? Future Oncol. 2014;10(7):1277-12797. doi:10.2217/fon.13.271
- Shaw E, Scott C, Souhami L, et al. Single dose radiosurgical treatment of recurrent previously irradiated primary brain tumors and brain metastases: final report of RTOG protocol 90-05. Int J Radiat Oncol Biol Phys. 2000;47(2):291-298. doi:10.1016/s0360-3016(99)00507-6
- Milano MT, Grimm J, Niemierko A, et al. Single- and multifraction stereotactic radiosurgery dose/volume tolerances of the brain. Int J Radiat Oncol Biol Phys. 2021;110(1):68-86. doi:10.1016/j.ijrobp.2020.08.013
- Id Said B, Chen H, Jerzak KJ, et al. Trastuzumab emtansine increases the risk of stereotactic radiosurgery-induced radionecrosis in HER2 + breast cancer. J Neurooncol. 2022;159(1):177-183. doi:10.1007/s11060-022-04055-y
- Carlson JA, Nooruddin Z, Rusthoven C, et al. Trastuzumab emtansine and stereotactic radiosurgery: an unexpected increase in clinically significant brain edema. Neuro Oncol. 2014;16(7):1006-1009. doi:10.1093/neuonc/not329
- Minniti G, Scaringi C, Paolini S, et al. Repeated stereotactic radiosurgery for patients with progressive brain metastases. J Neurooncol. 2016;126(1):91-97. doi:10.1007/s11060-015-1937-4
- Eaton DJ, Lee J, Paddick I. Stereotactic radiosurgery for multiple brain metastases: results of multicenter benchmark planning studies. Pract Radiat Oncol. 2018;8(4):e212-e220. doi:10.1016/j.prro.2017.12.011
- Milano MT, Soltys SG, Marks LB, et al. The art of radiation therapy: the necessary risk of radiation necrosis for durable control of brain metastases. Int J Radiat Oncol Biol Phys. 2023;115(2):294-296. doi:10.1016/j.ijrobp.2022.07.036
- Molenaar R, Wiggenraad R, Verbeek-de Kanter A, Walchenbach R, Vecht C. Relationship between volume, dose and local control in stereotactic radiosurgery of brain metastasis. Br J Neurosurg. 2009;23(2):170-178. doi:10.1080/02688690902755613
- Andrews DW, Scott CB, Sperduto PW, et al. Whole brain radiation therapy with or without stereotactic radiosurgery boost for patients with one to three brain metastases: phase III results of the RTOG 9508 randomised trial. Lancet. 2004;363(9422):1665-1672. doi:10.1016/S0140-6736(04)16250-8
- Scorsetti M, Navarria P, Cozzi L, et al. Radiosurgery of limited brain metastases from primary solid tumor: results of the randomized phase III trial (NCT02355613) comparing treatments executed with a specialized or a C-arm linac-based platform. Radiat Oncol. 2023;18(1):28. doi:10.1186/s13014-023-02216-5
- Bishop AJ, Tao R, Rebueno NC, et al. Outcomes for spine stereotactic body radiation therapy and an analysis of predictors of local recurrence. Int J Radiat Oncol Biol Phys. 2015;92(5):1016-1026. doi:10.1016/j.ijrobp.2015.03.037
- Lovelock DM, Zhang Z, Jackson A, et al. Correlation of local failure with measures of dose insufficiency in the high-dose single-fraction treatment of bony metastases. Int J Radiat Oncol Biol Phys. 2010;77(4):1282-1287. doi:10.1016/j.ijrobp.2009.10.003
- Grishchuk D, Dimitriadis A, Sahgal A, et al. ISRS technical guidelines for stereotactic radiosurgery: treatment of small brain metastases (≤1 cm in Diameter). Pract Radiat Oncol. 2023;13(3)183-194. doi:10.1016/j.prro.2022.10.013
- Shiau CY, Sneed PK, Shu HK, et al. Radiosurgery for brain metastases: relationship of dose and pattern of enhancement to local control. Int J Radiat Oncol Biol Phys. 1997;37(2):375-383. doi:10.1016/s0360-3016(96)00497-x
- Hasegawa T, Kondziolka D, Flickinger JC, Germanwala A, Lunsford LD. Brain metastases treated with radiosurgery alone: an alternative to whole brain radiotherapy? Neurosurgery. 2003;52(6):1318-26; discussion 1326. doi:10.1227/01.neu.0000064569.18914.de
- Lehrer EJ, Peterson JL, Zaorsky NG, et al. Single versus multifraction stereotactic radiosurgery for large brain metastases: an international meta-analysis of 24 trials. Int J Radiat Oncol Biol Phys. 2019;103(3):618-630. doi:10.1016/j.ijrobp.2018.10.038
- Akanda ZZ, Hong W, Nahavandi S, Haghighi N, Phillips C, Kok DL. Post-operative stereotactic radiosurgery following excision of brain metastases: a systematic review and meta-analysis. Radiother Oncol. 2020;142:27-35. doi:10.1016/j.radonc.2019.08.024
- Park HR, Park KW, Lee JM, et al. Frameless fractionated Gamma Knife radiosurgery with ICON™ for large metastatic brain tumors. J Korean Med Sci. 2019;34(8):e57. doi:10.3346/jkms.2019.34.e57
- Minniti G, Esposito V, Clarke E, et al. Multidose stereotactic radiosurgery (9 Gy × 3) of the postoperative resection cavity for treatment of large brain metastases. Int J Radiat Oncol Biol Phys. 2013;86(4):623-629. doi:10.1016/j.ijrobp.2013.03.037
- Minniti G, Scaringi C, Lanzetta G, et al. Comparative effectiveness of multi-fraction stereotactic radiosurgery for surgically resected or intact large brain metastases from non-small-cell lung cancer (NSCLC). Lung Cancer. 2019;132:119-125. doi:10.1016/j.lungcan.2019.04.021
- Kim KH, Kong DS, Cho KR, et al. Outcome evaluation of patients treated with fractionated Gamma Knife radiosurgery for large (> 3 cm) brain metastases: a dose-escalation study. J Neurosurg. 2019:1-10. doi:10.3171/2019.5.JNS19222
- Soltys S, Seiger K, Modlin L, et al. A phase I/II dose-escalation trial of 3-fraction stereotactic radiosurgery (srs) for large resection cavities of brain metastases. [Abstract]. American Society for Radiation Oncology 2015 Annual Meeting.
- Marcrom SR, McDonald AM, Thompson JW, et al. Fractionated stereotactic radiation therapy for intact brain metastases. Adv Radiat Oncol. 2017;2(4):564-571. doi:10.1016/j.adro.2017.07.006
- Minniti G, D’Angelillo RM, Scaringi C, et al. Fractionated stereotactic radiosurgery for patients with brain metastases. J Neurooncol. Apr 2014;117(2):295-301. doi:10.1007/s11060-014-1388-3
- Eaton B, Chen Z, Butker E, et al. Phase I dose escalation trial of hypofractionated radiosurgery for large intact or resected brain metastases. [Abstract]. American Society for Radiation Oncology 2021 Annual Meeting.
Citation
Rahimy E, Soltys SG. Stereotactic Radiosurgery for Brain Metastases: Review of Existing Data and Future Directions. Appl Radiat Oncol. 2023;(2):5-14.
July 4, 2023