Strategies to mitigate the effects of whole-brain radiation therapy on neurocognitive function in patients with brain metastases
Images
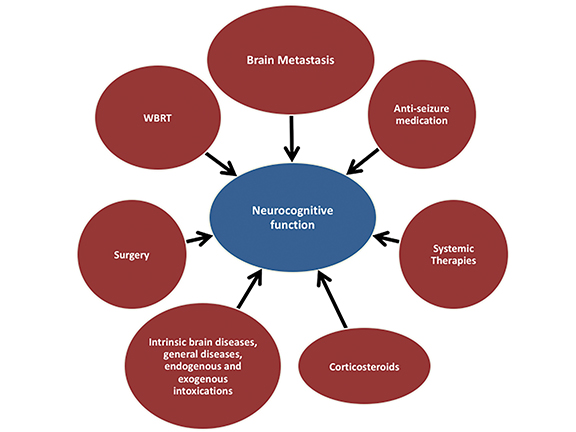
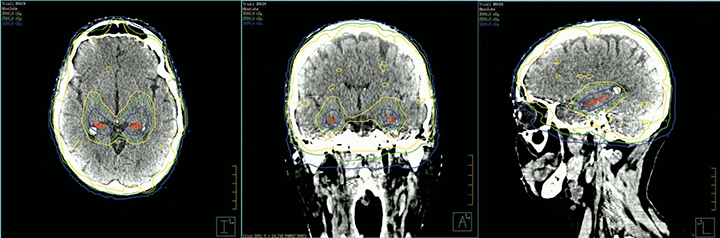
Brain metastases (BM) account for the vast majority of intracranial malignancies in adults, occurring in 20% to 40% of all patients with malignant tumors (mostly from lung and breast cancers).1,2 Due to improved local and systemic therapies and increased utilization of MR imaging, the incidence of BM is rising. Despite technological advances and more treatment options for these patients, unselected populations with BM continue to have a poor prognosis and survival.2,3
The use of prognostic systems such as the recursive partitioning analysis (RPA) and graded prognostic assessment (GPA) have helped to categorize BM patients based on several factors, such as age at diagnosis, Karnofsky Performance Status (KPS), status of the primary cancer, number of brain lesions, and absence or presence of extracranial disease.4,5 Disease-specific GPAs have been developed,6 including the incorporation of molecular markers.7 Nomograms have also been generated to provide individual survival estimates and help decide on treatment options.8
The most common treatment for patients with > 5 BM is whole-brain radiation therapy (WBRT). Currently, use of stereotactic radiosurgery, especially for patients with 1-4 lesions, and systemic approaches with or without ablative treatments, is increasing2,9 in part due to the toxicity associated with WBRT. In this review, we discuss strategies to mitigate the effects of WBRT on neurocognitive function in patients with BM.
Whole-brain Radiation Therapy (WBRT)
Since its inception in the 1950s, WBRT has been the primary treatment option for patients with BM.10 For decades, this treatment was considered the gold standard for patients with BM as it was easy to deliver, readily available, and effective at palliating neurologic signs and symptoms. Multiple phase III studies were conducted to determine the optimal dose and fractionation scheme of WBRT, with median survivals ranging 3 to 6 months.11-14
The results of the Quality of Life after Treatment for Brain Metastases (QUARTZ) trial have brought into question the use of WBRT for poor performance status patients with BM.15 In this study, patients with BM from nonsmall cell lung cancer were randomized to optimal supportive care vs. WBRT. The use of WBRT did not improve quality of life, overall survival, or decrease steroid use compared to best supportive care. However, the trial has been criticized because of poor survival in both arms (8-9 weeks), an unplanned early evaluation that biased results, and the high rate of steroid use in both arms (98% received at least 8 mg of daily dexamethasone), which may have impacted the EuroQol EQFD-3L quality of life metric used in the trial.
Neurocognitive Decline from WBRT
For years, toxicities from WBRT, including neurocognitive decline, were largely ignored given the poor outcomes associated with BM. A wide spectrum of WBRT-associated neurological impairments have been described,16-18 many of which are believed to have multifactorial causes, including radiation-induced injury, effects from tumors, and chemotherapy (Figure 1).
A phase III trial of WBRT vs. WBRT plus motexafin gadolinium, a radiation sensitizer, provided the first comprehensive assessment of neurocognitive changes in patients undergoing WBRT.19,20 Assessment was performed using the Hopkins Verbal Learning Test (HVLT) for immediate recall, delayed recall, and recognition; Controlled Oral Word Association (COWA) for verbal fluency; Trailmaking Test A for visual-motor scanning speed; Trailmaking Test B for executive function; and pegboard dominant hand and pegboard nondominant hand for fine motor skills. Of 401 enrolled patients, 90.5% had neurocognitive impairment prior to WBRT initiation. The addition of motexafin gadolinium did not result in a significant reduction in cognitive decline compared to WBRT alone; however, a subgroup analysis suggested that patients with NSCLC benefited from the standpoint of time to neurocognitive progression. Based on its design, the study was not able to identify neurocognitive changes attributable to WBRT;20 however, regression of brain metastases after WBRT was associated with improved survival and preserved neurocognitive function.21
Given concern for the effect of WBRT on neurocognitive function, the use of stereotactic radiosurgery (SRS) as a primary modality for BM treatment has increased. A phase III study performed at the University of Texas MD Anderson Cancer Center, Houston, reported by Chang and colleagues randomized patients to SRS plus WBRT compared to SRS alone and demonstrated greater decline in memory (as demonstrated by the Hopkins Verbal Learning Test-Revised [HVLT-R]) at 4 months) in patients who received WBRT.22 Other studies have demonstrated that the addition of WBRT to SRS in selected patients does not improve survival but does decrease distant brain failures, at the cost of decreased cognitive function and quality of life at 3 months.23-25 Despite these results, it is important to remember that a systematic review of neurocognitive effects of WBRT for newly diagnosed brain metastases reported that neurocognitive decline is predominant at 4 months, mild in severity (only 8% ≥ grade 2 on the SOMA-LENR scale), strongly dependent on brain metastases control, and partially resolved at a later time.26
Given increasing concern regarding the effect of WBRT on neurocognitive function, strategies to mitigate the effects of WBRT are an unmet need and are being actively explored. This article reviews approaches and rationale for avoiding or limiting damage to healthy brain, small and medium blood vessels, the hippocampus, and white matter.
Strategies to Mitigate WBRT Effects
The following strategies have shown promise for preventing neurocognitive decline: hippocampal avoidance (HA)-WBRT, the NMDA receptors antagonist memantine, renin angiotensin-aldosterone system (RAAS) blockers, donepezil / lithium, peroxisomal proliferator-activated receptor agonists, and use of SRS alone (the latter not addressed in this review). It is important to recognize that SRS alone is not appropriate in every case of BM, such as patients with multiple metastases (> 5 lesions), leptomeningeal disease, and small cell lung cancer histology; hence, strategies to mitigate effects of WBRT are essential.
Hippocampal avoidance (HA)-WBRT
The hippocampus plays an integral role in memory formation. Neural stem cells in the subgranular zone of the hippocampus are susceptible to radiation damage, which usually compromises memory function.27,28
The rationale of HA-WBRT arose from the observation that cognitive function deficits following WBRT correlated with hippocampal-related functions of memory, learning, and spatial processing. Based on this observation, two studies sought to determine if hippocampal sparing was feasible from the standpoint of hippocampal involvement by metastasis. Wan et al showed a 1.1% involvement rate (of more than 2,270 metastases) in hippocampal regions.29 In an analysis of 371 BM patients with a total of 1,133 tumors, Gondi et al found no hippocampal lesions; however, 9% of patients had tumors within 5 mm of the hippocampal regions.30
RTOG 0933, a phase II trial, analyzed the impact of HA-WBRT on declarative memory and used specific contouring guidelines for the subgranular zones of the hippocampi with a 5-mm expansion.31 Dose to 100% of the hippocampus was limited to 9 Gy with a maximum dose limited to 16 Gy. The remaining brain parenchyma received a dose of 30 Gy in 10 fractions. In this study, 42 patients showed a mean decline from baseline in HVLT delayed recall (HVLT-DR) of 7% at 4 months compared to 30% observed in a historical control treated with traditional WBRT (p = 0.0003). Of note, 8% is what one might expect for immediate memory deterioration with SRS alone. No decline on QoL was reported nor was < grade 4 toxicity.31 Figure 2 shows HA-WBRT using linear accelerator-based intensity-modulated radiation therapy (IMRT). Currently, two randomized trials, NRG CC001 and CC003, described later, are ongoing to confirm the results of RTOG 0933.
NMDA Receptor Antagonist (Memantine)
N-methyl-D-aspartate (NMDA) receptors in the hippocampus are activated by glutamate and play a role in learning and memory. Radiation therapy to the brain can overexcite these receptors, which alters the ratio of NMDA to GABA receptors, translating to possible neuronal cell death (excitotoxicity). Memantine is a noncompetitive NMDA receptor antagonist with proven efficacy to prevent receptor remodeling and preserve long-term potentiation in animal models and in vascular and Alzheimer’s dementia.32,33
The role of memantine was assessed by RTOG 0614, a phase III trial comparing WBRT (37.5 Gy in 15 fractions) plus memantine vs. WBRT plus placebo.34 This trial enrolled 554 patients with memantine administered within 3 days of starting WBRT and for the following 24 weeks, escalating to a final dose of 10 mg BID. At 24 weeks, a decline in delayed recall (HVLT-DR) was appreciated in patients receiving memantine. Unfortunately, the study was underpowered (35%), as only 149 patients were analyzable at 24 weeks due to early deaths in both arms, and this result was not statistically significant (p = 0.059). The addition of memantine did, however, demonstrate a longer time to cognitive decline with a probability of cognitive function failure at 24 weeks of 53.8% vs. 64.9% (p = 0.01).34 The authors of the study advocate for the routine use of memantine in patients receiving WBRT given the low toxicity and longer time to cognitive decline, despite not meeting the primary HVLT-DR endpoint. As part of the trial, a significant number of patients also enrolled on the translational section of the study in which apoE (Alzheimer’s gene) and inflammatory markers were measured. Results and correlation to outcome are expected later this year.
Renin Angiotensin System (RAS) Blockers
The Renin Angiotensin System (RAS) is known for having marked effects within organs as well as a systemic role in fluid balance. The local brain RAS is complex and involves maintenance of the blood-brain barrier, learning, memory spectrums, behaviors, and emotions.35 Preclinical models have demonstrated that the RAS may be involved in radiation-induced damage. The blockade of the RAS in irradiated rats has been shown to prevent radiation effects in lung and kidney tissues.36,37
Kim et al administered ramipril for 6 months to rats treated with brain RT (30 Gy), reporting a significant reduction in the demyelination of optic nerves and reduced severity of visual injury with the addition of Ramipril.38 Similarly, the administration of an angiotensin receptor blocker, L-158,809, to rats prior to and after 40 Gy WBRT prevented radiation-induced cognitive impairment.39
While RAS blockers have proven activity in modulating radiation-induced brain injury, their mechanism of action in the brain is poorly understood. However, based on their safety profile, widespread use, and potential benefits to neurocognitive function, they should be considered in future studies for patients receiving WBRT.40
Donepezil
Donepezil, an acetylcholinesterase inhibitor, is used to treat mild to moderate dementia in Alzheimer’s disease (AD). This drug enhances cholinergic neurotransmission by delaying breakdown of acetylcholine in synaptic clefts, a mechanism associated with memory.
In this context, improved cognitive function, mood and QoL were reported when donepezil was administrated for 24 weeks in a group of patients with primary brain tumors who had post-RT survival ≥ 6 months.41 Following these results, a randomized, double-blinded, placebo-controlled trial of donepezil and partial or WBRT was performed. 42
Rapp et al reported on 198 adult brain tumor survivors ≥ 6 months who received central nervous system (CNS) irradiation (WBRT or partial) to ≥ 30 Gy and were randomly assigned to receive 24 weeks of donepezil 5-10 mg per day or a placebo. Cognitive functioning was evaluated at baseline, 12 weeks, and 24 weeks with a battery of neuropsychological tests (cognitive composite score), which was completed by 74% of the participants. Treatment with donepezil did not significantly improve the overall composite score, but the donepezil group performed better than placebo on memory (recognition, p = .027; discrimination, p = .007), and motor speed and dexterity tests (p = .016). Significant interactions between pretreatment cognitive function and treatment were found for cognitive composite (p = .01), immediate recall (p = .05), delayed recall (p = .004), attention (p = .01), visual-motor skills (p = .02), and motor speed and dexterity (p < .001).42
While it appears donepezil has a role in treating cognitive impairment associated with brain cancer and its treatments, more studies are necessary to prove the value of this drug in this selected population.
Peroxisomal Proliferator-activated Receptor Agonists (PPAR)
Chronic inflammation has been implicated in the development and progression of radiation-induced late effects.43 This provides a rationale for the application of anti-inflammatory interventions to reduce radiation-induced brain injury. Peroxisomal proliferator-activated receptors (PPAR) α, β (δ), and γ are members of the nuclear hormone receptor superfamily of ligand-activated transcription factors that regulate gene expression.44 PPAR activation can affect anti-proliferative and anti-inflammatory cellular physiology. These effects are observed in many cell types, including brain glial cells and blood lymphocytes, cells whose activation contributes to the initiation and progression of damage occurring in neurological diseases such as AD and multiple sclerosis (MS).45
Animal models have demonstrated the impact of PPAR agonists to reduce radiation-therapy-related late cognitive effects. For example, administration of the anti-inflammatory peroxisomal proliferator-activated receptor γ (PPARγ) agonist, pioglitazone, to adult male rats was proven to substantially reduce radiation-induced cognitive impairment and similar results have been shown for the PPAR alpha agonist, fenofibrate.46,47 Furthermore, fenofibrate preserves hippocampal neurogenesis and inhibits microglial activation after WBRT, and protects cortical neurons from inflammatory mediators.48,49
Similar anti-inflammatory properties have been demonstrated with the PPARγ agonist, pioglitazone.50 Data shows promise for mitigating cognitive changes related to brain radiation therapy, and a phase 1 trial (NCT01151670) studying the side effects and optimal dose of pioglitazone hydrochloride in preventing radiation-induced cognitive dysfunction for both patients with BM and primary brain tumors was recently completed.
Ongoing Studies
The NRG has two ongoing studies to corroborate the results of RTOG 0614 and RTOG 0933. NRG-CC003 is a randomized phase II/III trial of prophylactic cranial irradiation (PCI) comparing WBRT to HA-WBRT in patients with extensive and limited-stage small-cell lung cancer who achieve a complete or partial response to chemotherapy. The randomized phase II trial is a noninferiority trial to determine whether the 12-month brain relapse rate following HA-PCI is noninferior compared to the rate after PCI. The phase III trial tests whether HA-PCI reduces the likelihood of a 6-month decline in HVLT-R delayed recall compared to PCI. Patients will be stratified by memantine use, stage (limited vs. extensive), and age (< 60 vs. > 60 years old).
NRG-CC001 is a randomized phase III trial of HA-WBRT plus memantine vs. WBRT (30 Gy in 10 fractions) plus memantine for patients with histologically or cytologically proven diagnosis of solid tumor malignancy within 5 years prior. This trial will determine whether use of HA-WBRT increases time to neurocognitive failure at months 2, 4, 6 and 12 as measured by neurocognitive decline on a battery of tests compared to WBRT. Prior therapy for brain metastasis, including radiosurgery and surgical resection, is allowed.
Conclusion
Treatments affecting neurocognitive function are of major concern for patients, their families, and physicians. It is, therefore, of paramount importance that treatment strategies for BM balance tumor control and survival with preservation of cognitive function, which impacts quality of life. The etiology of neurocognitive decline in cancer patients with BM is multifactorial and includes the tumors themselves, systemic agents, and the effects of WBRT.
Current approaches to reduce the effects of WBRT on neurocognitive function in patients with BM include avoidance of WBRT, implementation of HA-WBRT, and prophylactic use of the NMDA receptor antagonist memantine. Both HA-WBRT and memantine use are being investigated in ongoing NRG studies. Other promising strategies include RAS blockers, acetylcholinesterase inhibitors, and peroxisomal proliferator-activated receptor agonists.
We strongly encourage enrollment in ongoing and future trials that investigate strategies to mitigate the effects of WBRT on neurocognitive function in patients with BM.
References
- Patchell RA. The management of brain metastases. Cancer Treat Rev. 2003;29(6):533-540.
- Moraes FY, Taunk NK, Marta GN, et al. The rationale for targeted therapies and stereotactic radiosurgery in the treatment of brain metastases. Oncologist. 2016;21(2):244-251.
- Moraes FY, Bonifacio LA, Marta GN, et al. Hierarchy of evidence referring to the central nervous system in a high-impact radiation oncology journal: a 10-year assessment. Descriptive critical appraisal study. São Paulo Med J. 2015; 133(4):307-313.
- Gaspar L, Scott C, Rotman M, et al. Recursive partitioning analysis (RPA) of prognostic factors in three Radiation Therapy Oncology Group (RTOG) brain metastases trials. Int J Radiat Oncol Biol Phys. 1997;37(4):745-751.
- Sperduto PW, Berkey B, Gaspar LE, et al. A new prognostic index and comparison to three other indices for patients with brain metastases: an analysis of 1,960 patients in the RTOG database. Int J Radiat Oncol Biol Phys. 2008;70(2):510-514.
- Sperduto PW, Chao ST, Sneed PK, et al. Diagnosis-specific prognostic factors, indexes, and treatment outcomes for patients with newly diagnosed brain metastases: a multi-institutional analysis of 4,259 patients. Int J Radiat Oncol Biol Phys. 2010;77(3):655-661.
- Sperduto PW, Yang TJ, Beal K, et al. Estimating survival in patients with lung cancer and brain metastases: an update of the graded prognostic assessment for lung cancer using molecular markers (Lung-molGPA). JAMA Oncol. 2016 Nov 17; doi: 10.1001/jamaoncol.2016.3834. [Epub ahead of print.]
- Barnholtz-Sloan JS, Yu C, Sloan AE, et al. A nomogram for individualized estimation of survival among patients with brain metastasis. Neuro-Oncol. 2012;14(7):910-918.
- Sahgal A, Aoyama H, Kocher M, et al. Phase 3 trials of stereotactic radiosurgery with or without whole-brain radiation therapy for 1 to 4 brain metastases: individual patient data meta-analysis. Int J Radiat Oncol Biol Phys. 2015;91(4):710-717.
- Chao JH, Phillips R, Nickson JJ. Roentgen-ray therapy of cerebral metastases. Cancer. 1954;7(4):682-689.
- Borgelt B, Gelber R, Kramer S, et al. The palliation of brain metastases: final results of the first two studies by the Radiation Therapy Oncology Group. Int J Radiat Oncol Biol Phys. 1980;6(1):1-9.
- Borgelt B, Gelber R, Larson M, et al. Ultra-rapid high dose irradiation schedules for the palliation of brain metastases: final results of the first two studies by the Radiation Therapy Oncology Group. Int J Radiat Oncol Biol Phys. 1981;7(12):1633-1638.
- Kurtz JM, Gelber R, Brady LW, et al. The palliation of brain metastases in a favorable patient population: a randomized clinical trial by the Radiation Therapy Oncology Group. Int J Radiat Oncol Biol Phys. 1981;7(7):891-895.
- Murray KJ, Scott C, Greenberg HM, et al. A randomized phase III study of accelerated hyperfractionation versus standard in patients with unresected brain metastases: a report of the Radiation Therapy Oncology Group (RTOG) 9104. Int J Radiat Oncol Biol Phys. 1997;39(3):571-574.
- Mulvenna P, Nankivell M, Barton R, et al. Dexamethasone and supportive care with or without whole brain radiotherapy in treating patients with non-small cell lung cancer with brain metastases unsuitable for resection or stereotactic radiotherapy (QUARTZ): results from a phase 3, non-inferiority, randomised trial. Lancet Lond Engl. 2016;388(10055):2004-2014.
- Lee YW, Cho HJ, Lee WH, Sonntag WE. Whole brain radiation-induced cognitive impairment: pathophysiological mechanisms and therapeutic targets. Biomol Ther. 2012;20(4):357-370.
- Gerstenecker A, Nabors LB, Meneses K, et al. Cognition in patients with newly diagnosed brain metastasis: profiles and implications. J Neurooncol. 2014;120(1):179-185.
- Owonikoko TK, Arbiser J, Zelnak A, et al. Current approaches to the treatment of metastatic brain tumours. Nat Rev Clin Oncol. 2014;11(4):203-222.
- Mehta MP, Rodrigus P, Terhaard CHJ, et al. Survival and neurologic outcomes in a randomized trial of motexafin gadolinium and whole-brain radiation therapy in brain metastases. J Clin Oncol. 2003;21(13):2529-2536.
- Meyers CA, Smith JA, Bezjak A, et al. Neurocognitive function and progression in patients with brain metastases treated with whole-brain radiation and motexafin gadolinium: results of a randomized phase III trial. J Clin Oncol. 2004;22(1):157-165.
- Li J, Bentzen SM, Renschler M, Mehta MP. Regression after whole-brain radiation therapy for brain metastases correlates with survival and improved neurocognitive function. J Clin Oncol. 2007;25(10):1260-1266.
- Chang EL, Wefel JS, Hess KR, et al. Neurocognition in patients with brain metastases treated with radiosurgery or radiosurgery plus whole-brain irradiation: a randomised controlled trial. Lancet Oncol. 2009;10(11):1037-1044.
- Aoyama H, Shirato H, Tago M, et al. Stereotactic radiosurgery plus whole-brain radiation therapy vs stereotactic radiosurgery alone for treatment of brain metastases: a randomized controlled trial. JAMA. 2006;295(21):2483–2491.
- Kocher M, Soffietti R, Abacioglu U, et al. Adjuvant whole-brain radiotherapy versus observation after radiosurgery or surgical resection of one to three cerebral metastases: results of the EORTC 22952-26001 study. J Clin Oncol. 2011;29(2):
- 134-141.
- Brown PD, Jaeckle K, Ballman KV, et al. Effect of radiosurgery alone vs radiosurgery with whole brain radiation therapy on cognitive function in patients with 1 to 3 brain metastases: a randomized clinical trial. JAMA. 2016 ;316(4):401-409.
- Tallet AV, Azria D, Barlesi F, et al. Neurocognitive function impairment after whole brain radiotherapy for brain metastases: actual assessment. Radiat Oncol. 2012 May 28;7:77.
- Raber J, Rola R, LeFevour A, et al. Radiation-induced cognitive impairments are associated with changes in indicators of hippocampal neurogenesis. Radiat Res. 2004;162(1):39-47.
- Rola R, Raber J, Rizk A, et al. Radiation-induced impairment of hippocampal neurogenesis is associated with cognitive deficits in young mice. Exp Neurol. 2004;88(2):316-330.
- Wan J-F, Zhang S-J, Wang L, Zhao K-L. Implications for preserving neural stem cells in whole brain radiotherapy and prophylactic cranial irradiation: a review of 2270 metastases in 488 patients. J Radiat Res (Tokyo). 2013;54(2):285-291.
- Gondi V, Tome WA, Marsh J, et al. Estimated risk of perihippocampal disease progression after hippocampal avoidance during whole-brain radiotherapy: safety profile for RTOG 0933. Radiother Oncol. 2010;95(3):327-331.
- Gondi V, Pugh SL, Tome WA, et al. Preservation of memory with conformal avoidance of the hippocampal neural stem-cell compartment during whole-brain radiotherapy for brain metastases (RTOG 0933): a phase II multi-institutional trial. J Clin Oncol. 2014;32(34):
- 3810-3816.
- Wu PH, Coultrap S, Pinnix C, et al. Radiation induces acute alterations in neuronal function. PLoS ONE [Internet]. 2012 May 25;7(5). http://www.ncbi.nlm.nih.gov/pmc/articles/PMC3360766/. Accessed January 23, 2017.
- Wilcock G, Möbius HJ, Stöffler A, MMM 500 group. A double-blind, placebo-controlled multicentre study of memantine in mild to moderate vascular dementia (MMM500). Int Clin Psychopharmacol. 2002;17(6):297-305.
- Brown PD, Pugh S, Laack NN, et al. Memantine for the prevention of cognitive dysfunction in patients receiving whole-brain radiotherapy: a randomized, double-blind, placebo-controlled trial. Neuro-Oncol. 2013;15(10):1429-1437.
- Paul M, Poyan Mehr A, Kreutz R. Physiology of local renin-angiotensin systems. Physiol Rev. 2006;86(3):747-803.
- Moulder JE, Cohen EP, Fish BL. Captopril and losartan for mitigation of renal injury caused by single-dose total-body irradiation. Radiat Res. 2011;175(1):29-36.
- Ghosh SN, Zhang R, Fish BL, et al. Renin-Angiotensin system suppression mitigates experimental radiation pneumonitis. Int J Radiat Oncol Biol Phys. 2009;75(5):1528–1536.
- Kim JH, Brown SL, Kolozsvary A, et al. Modification of radiation injury by ramipril, inhibitor of angiotensin-converting enzyme, on optic neuropathy in the rat. Radiat Res. 2004;161(2):137-142.
- Robbins ME, Payne V, Tommasi E, et al. The AT1 receptor antagonist, L-158,809, prevents or ameliorates fractionated whole-brain irradiation-induced cognitive impairment. Int J Radiat Oncol Biol Phys. 2009;73(2):499-505.
- Belka C, Budach W, Kortmann RD, Bamberg M. Radiation induced CNS toxicity—molecular and cellular mechanisms. Br J Cancer. 2001 Nov 2;85(9):
- 1233-1239.
- Shaw EG, Rosdhal R, D’Agostino RB, et al. Phase II study of donepezil in irradiated brain tumor patients: effect on cognitive function, mood, and quality of life. J Clin Oncol. 2006;24(9):1415-1420.
- Rapp SR, Case LD, Peiffer A, et al. Donepezil for irradiated brain tumor survivors: a phase iii randomized placebo-controlled clinical trial. J Clin Oncol. 2015;33(15):1653-1659.
- Robbins MEC, Zhao W. Chronic oxidative stress and radiation-induced late normal tissue injury: a review. Int J Radiat Biol. 2004;80(4):251-259.
- Lemberger T, Desvergne B, Wahli W. Peroxisome proliferator-activated receptors: a nuclear receptor signaling pathway in lipid physiology. Annu Rev Cell Dev Biol. 1996;12:335-363.
- Feinstein DL. Therapeutic potential of peroxisome proliferator-activated receptor agonists for neurological disease. Diabetes Technol Ther. 2003;5(1):67-73.
- Zhao W, Payne V, Tommasi E, et al. Administration of the peroxisomal proliferator-activated receptor gamma agonist pioglitazone during fractionated brain irradiation prevents radiation-induced cognitive impairment. Int J Radiat Oncol Biol Phys. 2007;67(1):6-9.
- Greene-Schloesser D, Payne V, Peiffer AM, et al. The peroxisomal proliferator-activated receptor (PPAR) α agonist, fenofibrate, prevents fractionated whole-brain irradiation-induced cognitive impairment. Radiat Res. 2014;181(1):33-44.
- Ramanan S, Kooshki M, Zhao W, et al. The PPARalpha agonist fenofibrate preserves hippocampal neurogenesis and inhibits microglial activation after whole-brain irradiation. Int J Radiat Oncol Biol Phys. 2009;75(3):870-877.
- Gray E, Ginty M, Kemp K, et al. Peroxisome proliferator-activated receptor-α agonists protect cortical neurons from inflammatory mediators and improve peroxisomal Gray E, Ginty M, Kemp K, et al. The PPAR-γ agonist pioglitazone protects cortical neurons from inflammatory mediators via improvement in peroxisomal function. J Neuroinflammation. 2012;9:63.
Citation
Moraes FY, Shultz DB, Murphy ES, Khuntia D, Chao ST, Suh JH. Strategies to mitigate the effects of whole-brain radiation therapy on neurocognitive function in patients with brain metastases. Appl Radiat Oncol. 2017;(1):6-10.
March 8, 2017